Chimeric Glutamate Receptor Subunits Reveal the Transmembrane Domain Is Sufficient for NMDA Receptor Pore Properties but Some Positive Allosteric Modulators Require Additional Domains
- PMID: 27559165
- PMCID: PMC4995299
- DOI: 10.1523/JNEUROSCI.0345-16.2016
Chimeric Glutamate Receptor Subunits Reveal the Transmembrane Domain Is Sufficient for NMDA Receptor Pore Properties but Some Positive Allosteric Modulators Require Additional Domains
Abstract
NMDA receptors are ligand-gated ion channels that underlie transmission at excitatory synapses and play an important role in regulating synaptic strength and stability. Functional NMDA receptors require two copies of the GluN1 subunit coassembled with GluN2 (and/or GluN3) subunits into a heteromeric tetramer. A diverse array of allosteric modulators can upregulate or downregulate NMDA receptor activity. These modulators include both synthetic compounds and endogenous modulators, such as cis-unsaturated fatty acids, 24(S)-hydroxycholesterol, and various neurosteroids. To evaluate the structural requirements for the formation and allosteric modulation of NMDA receptor pores, we have replaced portions of the rat GluN1, GluN2A, and GluN2B subunits with homologous segments from the rat GluK2 kainate receptor subunit. Our results with these chimeric constructs show that the NMDA receptor transmembrane domain is sufficient to account for most pore properties, but that regulation by some allosteric modulators requires additional cytoplasmic or extracellular domains.
Significance statement: Glutamate receptors mediate excitatory synaptic transmission by forming cation channels through the membrane that open upon glutamate binding. Although many compounds have been identified that regulate glutamate receptor activity, in most cases the detailed mechanisms that underlie modulation are poorly understood. To identify what parts of the receptor are essential for pore formation and sensitivity to allosteric modulators, we generated chimeric subunits that combined segments from NMDA and kainate receptors, subtypes with distinct pharmacological profiles. Surprisingly, our results identify separate domain requirements for allosteric potentiation of NMDA receptor pores by pregnenolone sulfate, 24(S)-hydroxycholesterol, and docosahexaenoic acid, three endogenous modulators derived from membrane constituents. Understanding where and how these compounds act on NMDA receptors should aid in designing better therapeutic agents.
Keywords: carboxy terminal domain; docosahexaenoic acid; palmitoylation.
Copyright © 2016 the authors 0270-6474/16/368815-11$15.00/0.
Figures
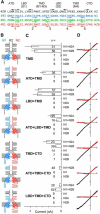
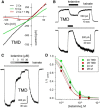
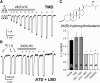
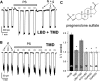
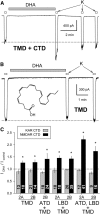
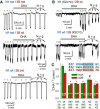
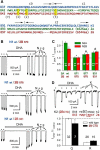
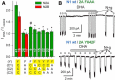
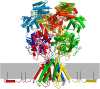
Similar articles
-
Arrangement of subunits in functional NMDA receptors.J Neurosci. 2011 Aug 3;31(31):11295-304. doi: 10.1523/JNEUROSCI.5612-10.2011. J Neurosci. 2011. PMID: 21813689 Free PMC article.
-
Different structural requirements for functional ion pore transplantation suggest different gating mechanisms of NMDA and kainate receptors.J Neurochem. 2008 Oct;107(2):453-65. doi: 10.1111/j.1471-4159.2008.05623.x. Epub 2008 Aug 14. J Neurochem. 2008. PMID: 18710418
-
Negative allosteric modulation of GluN1/GluN3 NMDA receptors.Neuropharmacology. 2020 Oct 1;176:108117. doi: 10.1016/j.neuropharm.2020.108117. Epub 2020 May 7. Neuropharmacology. 2020. PMID: 32389749 Free PMC article.
-
Diverse modes of NMDA receptor positive allosteric modulation: Mechanisms and consequences.Neuropharmacology. 2017 Jan;112(Pt A):34-45. doi: 10.1016/j.neuropharm.2016.07.037. Epub 2016 Jul 30. Neuropharmacology. 2017. PMID: 27484578 Review.
-
Molecular basis of NMDA receptor functional diversity.Eur J Neurosci. 2011 Apr;33(8):1351-65. doi: 10.1111/j.1460-9568.2011.07628.x. Epub 2011 Mar 14. Eur J Neurosci. 2011. PMID: 21395862 Review.
Cited by
-
Positive and Negative Allosteric Modulators of N-Methyl-d-aspartate (NMDA) Receptors: Structure-Activity Relationships and Mechanisms of Action.J Med Chem. 2019 Jan 10;62(1):3-23. doi: 10.1021/acs.jmedchem.7b01640. Epub 2018 Mar 5. J Med Chem. 2019. PMID: 29446949 Free PMC article. Review.
-
Divergent roles of a peripheral transmembrane segment in AMPA and NMDA receptors.J Gen Physiol. 2017 Jun 5;149(6):661-680. doi: 10.1085/jgp.201711762. Epub 2017 May 15. J Gen Physiol. 2017. PMID: 28507080 Free PMC article.
-
The NMDA receptor intracellular C-terminal domains reciprocally interact with allosteric modulators.Biochem Pharmacol. 2019 Jan;159:140-153. doi: 10.1016/j.bcp.2018.11.018. Epub 2018 Nov 29. Biochem Pharmacol. 2019. PMID: 30503374 Free PMC article.
-
Palmitoylation Controls NMDA Receptor Function and Steroid Sensitivity.J Neurosci. 2021 Mar 10;41(10):2119-2134. doi: 10.1523/JNEUROSCI.2654-20.2021. Epub 2021 Feb 1. J Neurosci. 2021. PMID: 33526476 Free PMC article.
-
Synthesis and evaluation of photoaffinity labeling reagents for identifying binding sites of sulfated neurosteroids on NMDA and GABAA receptors.RSC Adv. 2024 Nov 13;14(49):36352-36369. doi: 10.1039/d4ra07074g. eCollection 2024 Nov 11. RSC Adv. 2024. PMID: 39539530 Free PMC article.
References
-
- Collingridge GL, Volianskis A, Bannister N, France G, Hanna L, Mercier M, Tidball P, Fang G, Irvine MW, Costa BM, Monaghan DT, Bortolotto ZA, Molnár E, Lodge D, Jane DE. The NMDA receptor as a target for cognitive enhancement. Neuropharmacology. 2013;64:13–26. doi: 10.1016/j.neuropharm.2012.06.051. - DOI - PMC - PubMed
Publication types
MeSH terms
Substances
Associated data
- Actions
- Actions
Grants and funding
LinkOut - more resources
Full Text Sources
Other Literature Sources