Tropomodulin 1 Regulation of Actin Is Required for the Formation of Large Paddle Protrusions Between Mature Lens Fiber Cells
- PMID: 27537257
- PMCID: PMC4986768
- DOI: 10.1167/iovs.16-19949
Tropomodulin 1 Regulation of Actin Is Required for the Formation of Large Paddle Protrusions Between Mature Lens Fiber Cells
Abstract
Purpose: To elucidate the proteins required for specialized small interlocking protrusions and large paddle domains at lens fiber cell tricellular junctions (vertices), we developed a novel method to immunostain single lens fibers and studied changes in cell morphology due to loss of tropomodulin 1 (Tmod1), an F-actin pointed end-capping protein.
Methods: We investigated F-actin and F-actin-binding protein localization in interdigitations of Tmod1+/+ and Tmod1-/- single mature lens fibers.
Results: F-actin-rich small protrusions and large paddles were present along cell vertices of Tmod1+/+ mature fibers. In contrast, Tmod1-/- mature fiber cells lack normal paddle domains, while small protrusions were unaffected. In Tmod1+/+ mature fibers, Tmod1, β2-spectrin, and α-actinin are localized in large puncta in valleys between paddles; but in Tmod1-/- mature fibers, β2-spectrin was dispersed while α-actinin was redistributed at the base of small protrusions and rudimentary paddles. Fimbrin and Arp3 (actin-related protein 3) were located in puncta at the base of small protrusions, while N-cadherin and ezrin outlined the cell membrane in both Tmod1+/+ and Tmod1-/- mature fibers.
Conclusions: These results suggest that distinct F-actin organizations are present in small protrusions versus large paddles. Formation and/or maintenance of large paddle domains depends on a β2-spectrin-actin network stabilized by Tmod1. α-Actinin-crosslinked F-actin bundles are enhanced in absence of Tmod1, indicating altered cytoskeleton organization. Formation of small protrusions is likely facilitated by Arp3-branched and fimbrin-bundled F-actin networks, which do not depend on Tmod1. This is the first work to reveal the F-actin-associated proteins required for the formation of paddles between lens fibers.
Figures
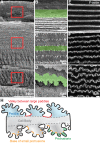
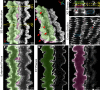
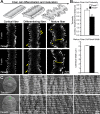
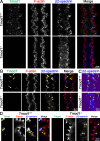
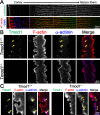
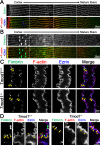
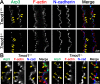
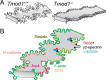
Comment in
-
New Insight Into the Protrusions and Paddles That Link Lens Fiber Cells.Invest Ophthalmol Vis Sci. 2016 Aug 1;57(10):4100. doi: 10.1167/iovs.16-20340. Invest Ophthalmol Vis Sci. 2016. PMID: 27537258 No abstract available.
Similar articles
-
Tropomodulin1 is required for membrane skeleton organization and hexagonal geometry of fiber cells in the mouse lens.J Cell Biol. 2009 Sep 21;186(6):915-28. doi: 10.1083/jcb.200905065. Epub 2009 Sep 14. J Cell Biol. 2009. PMID: 19752024 Free PMC article.
-
Tmod1 and CP49 synergize to control the fiber cell geometry, transparency, and mechanical stiffness of the mouse lens.PLoS One. 2012;7(11):e48734. doi: 10.1371/journal.pone.0048734. Epub 2012 Nov 7. PLoS One. 2012. PMID: 23144950 Free PMC article.
-
Breakdown of interlocking domains may contribute to formation of membranous globules and lens opacity in ephrin-A5(-/-) mice.Exp Eye Res. 2016 Apr;145:130-139. doi: 10.1016/j.exer.2015.11.017. Epub 2015 Nov 28. Exp Eye Res. 2016. PMID: 26643403 Free PMC article.
-
Capping actin filament growth: tropomodulin in muscle and nonmuscle cells.Soc Gen Physiol Ser. 1997;52:79-89. Soc Gen Physiol Ser. 1997. PMID: 9210222 Review.
-
The lens actin filament cytoskeleton: Diverse structures for complex functions.Exp Eye Res. 2017 Mar;156:58-71. doi: 10.1016/j.exer.2016.03.005. Epub 2016 Mar 10. Exp Eye Res. 2017. PMID: 26971460 Free PMC article. Review.
Cited by
-
The significance of growth shells in development of symmetry, transparency, and refraction of the human lens.Front Ophthalmol (Lausanne). 2024 Jul 19;4:1434327. doi: 10.3389/fopht.2024.1434327. eCollection 2024. Front Ophthalmol (Lausanne). 2024. PMID: 39100140 Free PMC article. Review.
-
Eph-ephrin Signaling Affects Eye Lens Fiber Cell Intracellular Voltage and Membrane Conductance.Front Physiol. 2021 Nov 25;12:772276. doi: 10.3389/fphys.2021.772276. eCollection 2021. Front Physiol. 2021. PMID: 34899394 Free PMC article.
-
EphA2 and Ephrin-A5 Guide Eye Lens Suture Alignment and Influence Whole Lens Resilience.Invest Ophthalmol Vis Sci. 2021 Dec 1;62(15):3. doi: 10.1167/iovs.62.15.3. Invest Ophthalmol Vis Sci. 2021. PMID: 34854885 Free PMC article.
-
Roles of Eph-Ephrin Signaling in the Eye Lens Cataractogenesis, Biomechanics, and Homeostasis.Front Cell Dev Biol. 2022 Feb 28;10:852236. doi: 10.3389/fcell.2022.852236. eCollection 2022. Front Cell Dev Biol. 2022. PMID: 35295853 Free PMC article. Review.
-
Age-related changes in eye lens biomechanics, morphology, refractive index and transparency.Aging (Albany NY). 2019 Dec 16;11(24):12497-12531. doi: 10.18632/aging.102584. Epub 2019 Dec 16. Aging (Albany NY). 2019. PMID: 31844034 Free PMC article.
References
-
- Lovicu FJ,, Robinson ML. Development of the Ocular Lens. Cambridge UK, New York: Cambridge University Press; 2004: xv, 398.
-
- Kuszak JR,, Zoltoski RK,, Sivertson C. Fibre cell organization in crystalline lenses. Exp Eye Res. 2004; 78: 673–687. - PubMed
-
- Kuszak JR,, Mazurkiewicz M,, Jison L,, Madurski A,, Ngando A,, Zoltoski RK. Quantitative analysis of animal model lens anatomy: accommodative range is related to fiber structure and organization. Vet Ophthalmol. 2006; 9: 266–280. - PubMed
-
- Piatigorsky J. Lens differentiation in vertebrates. A review of cellular and molecular features. Differentiation. 1981; 19: 134–153. - PubMed
Publication types
MeSH terms
Substances
Grants and funding
LinkOut - more resources
Full Text Sources
Other Literature Sources
Molecular Biology Databases
Research Materials
Miscellaneous