Structural conservation in the template/pseudoknot domain of vertebrate telomerase RNA from teleost fish to human
- PMID: 27531956
- PMCID: PMC5024593
- DOI: 10.1073/pnas.1607411113
Structural conservation in the template/pseudoknot domain of vertebrate telomerase RNA from teleost fish to human
Abstract
Telomerase is an RNA-protein complex that includes a unique reverse transcriptase that catalyzes the addition of single-stranded telomere DNA repeats onto the 3' ends of linear chromosomes using an integral telomerase RNA (TR) template. Vertebrate TR contains the template/pseudoknot (t/PK) and CR4/5 domains required for telomerase activity in vitro. All vertebrate pseudoknots include two subdomains: P2ab (helices P2a and P2b with a 5/6-nt internal loop) and the minimal pseudoknot (P2b-P3 and associated loops). A helical extension of P2a, P2a.1, is specific to mammalian TR. Using NMR, we investigated the structures of the full-length TR pseudoknot and isolated subdomains in Oryzias latipes (Japanese medaka fish), which has the smallest vertebrate TR identified to date. We determined the solution NMR structure and studied the dynamics of medaka P2ab, and identified all base pairs and tertiary interactions in the minimal pseudoknot. Despite differences in length and sequence, the structure of medaka P2ab is more similar to human P2ab than predicted, and the medaka minimal pseudoknot has the same tertiary interactions as the human pseudoknot. Significantly, although P2a.1 is not predicted to form in teleost fish, we find that it forms in the full-length pseudoknot via an unexpected hairpin. Model structures of the subdomains are combined to generate a model of t/PK. These results provide evidence that the architecture for the vertebrate t/PK is conserved from teleost fish to human. The organization of the t/PK on telomerase reverse transcriptase for medaka and human is modeled based on the cryoEM structure of Tetrahymena telomerase, providing insight into function.
Keywords: NMR; RNA structure; RNP; TERT; medaka.
Conflict of interest statement
The authors declare no conflict of interest.
Figures
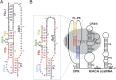
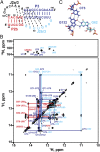


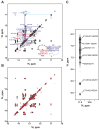
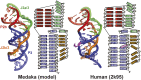
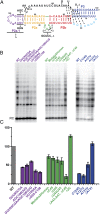
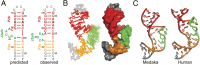
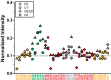
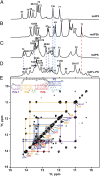
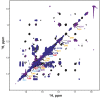
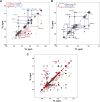
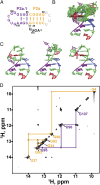
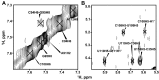
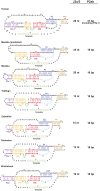
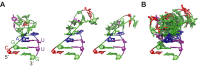
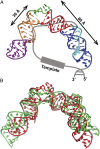
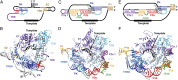
Similar articles
-
Structure and sequence elements of the CR4/5 domain of medaka telomerase RNA important for telomerase function.Nucleic Acids Res. 2014 Mar;42(5):3395-408. doi: 10.1093/nar/gkt1276. Epub 2013 Dec 11. Nucleic Acids Res. 2014. PMID: 24335084 Free PMC article.
-
Structure and function of the smallest vertebrate telomerase RNA from teleost fish.J Biol Chem. 2008 Jan 25;283(4):2049-59. doi: 10.1074/jbc.M708032200. Epub 2007 Nov 26. J Biol Chem. 2008. PMID: 18039659
-
Structurally conserved five nucleotide bulge determines the overall topology of the core domain of human telomerase RNA.Proc Natl Acad Sci U S A. 2010 Nov 2;107(44):18761-8. doi: 10.1073/pnas.1013269107. Epub 2010 Oct 21. Proc Natl Acad Sci U S A. 2010. PMID: 20966348 Free PMC article.
-
Structure and function of telomerase RNA.Curr Opin Struct Biol. 2006 Jun;16(3):307-18. doi: 10.1016/j.sbi.2006.05.005. Epub 2006 May 18. Curr Opin Struct Biol. 2006. PMID: 16713250 Review.
-
Evolutionary perspectives of telomerase RNA structure and function.RNA Biol. 2016 Aug 2;13(8):720-32. doi: 10.1080/15476286.2016.1205768. Epub 2016 Jun 30. RNA Biol. 2016. PMID: 27359343 Free PMC article. Review.
Cited by
-
RNAs as Regulators of Cellular Matchmaking.Front Mol Biosci. 2021 Apr 9;8:634146. doi: 10.3389/fmolb.2021.634146. eCollection 2021. Front Mol Biosci. 2021. PMID: 33898516 Free PMC article. Review.
-
Characterisation of the Arabidopsis thaliana telomerase TERT-TR complex.Plant Mol Biol. 2024 May 14;114(3):56. doi: 10.1007/s11103-024-01461-w. Plant Mol Biol. 2024. PMID: 38743198 Free PMC article.
-
Structure of active human telomerase with telomere shelterin protein TPP1.Nature. 2022 Apr;604(7906):578-583. doi: 10.1038/s41586-022-04582-8. Epub 2022 Apr 13. Nature. 2022. PMID: 35418675 Free PMC article.
-
Telomerase structures and regulation: shedding light on the chromosome end.Curr Opin Struct Biol. 2019 Apr;55:185-193. doi: 10.1016/j.sbi.2019.04.009. Epub 2019 Jun 12. Curr Opin Struct Biol. 2019. PMID: 31202023 Free PMC article. Review.
-
Structural Biology of Telomerase.Cold Spring Harb Perspect Biol. 2019 Dec 2;11(12):a032383. doi: 10.1101/cshperspect.a032383. Cold Spring Harb Perspect Biol. 2019. PMID: 31451513 Free PMC article. Review.
References
-
- Shay JW, Wright WE. Hayflick, his limit, and cellular ageing. Nat Rev Mol Cell Biol. 2000;1(1):72–76. - PubMed
-
- Harley CB. Telomerase and cancer therapeutics. Nat Rev Cancer. 2008;8(3):167–179. - PubMed
-
- Shay JW, Wright WE. Telomerase therapeutics for cancer: Challenges and new directions. Nat Rev Drug Discov. 2006;5(7):577–584. - PubMed
Publication types
MeSH terms
Substances
Associated data
- Actions
Grants and funding
LinkOut - more resources
Full Text Sources
Other Literature Sources
Research Materials