Microfluidic blood-brain barrier model provides in vivo-like barrier properties for drug permeability screening
- PMID: 27399645
- PMCID: PMC6650146
- DOI: 10.1002/bit.26045
Microfluidic blood-brain barrier model provides in vivo-like barrier properties for drug permeability screening
Abstract
Efficient delivery of therapeutics across the neuroprotective blood-brain barrier (BBB) remains a formidable challenge for central nervous system drug development. High-fidelity in vitro models of the BBB could facilitate effective early screening of drug candidates targeting the brain. In this study, we developed a microfluidic BBB model that is capable of mimicking in vivo BBB characteristics for a prolonged period and allows for reliable in vitro drug permeability studies under recirculating perfusion. We derived brain microvascular endothelial cells (BMECs) from human induced pluripotent stem cells (hiPSCs) and cocultured them with rat primary astrocytes on the two sides of a porous membrane on a pumpless microfluidic platform for up to 10 days. The microfluidic system was designed based on the blood residence time in human brain tissues, allowing for medium recirculation at physiologically relevant perfusion rates with no pumps or external tubing, meanwhile minimizing wall shear stress to test whether shear stress is required for in vivo-like barrier properties in a microfluidic BBB model. This BBB-on-a-chip model achieved significant barrier integrity as evident by continuous tight junction formation and in vivo-like values of trans-endothelial electrical resistance (TEER). The TEER levels peaked above 4000 Ω · cm2 on day 3 on chip and were sustained above 2000 Ω · cm2 up to 10 days, which are the highest sustained TEER values reported in a microfluidic model. We evaluated the capacity of our microfluidic BBB model to be used for drug permeability studies using large molecules (FITC-dextrans) and model drugs (caffeine, cimetidine, and doxorubicin). Our analyses demonstrated that the permeability coefficients measured using our model were comparable to in vivo values. Our BBB-on-a-chip model closely mimics physiological BBB barrier functions and will be a valuable tool for screening of drug candidates. The residence time-based design of a microfluidic platform will enable integration with other organ modules to simulate multi-organ interactions on drug response. Biotechnol. Bioeng. 2017;114: 184-194. © 2016 Wiley Periodicals, Inc.
Keywords: TEER; blood-brain barrier; human iPS cells; organ on a chip; permeability.
© 2016 Wiley Periodicals, Inc.
Figures
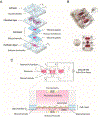
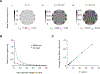
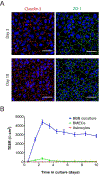
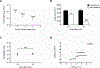
Similar articles
-
Characterization of a microfluidic in vitro model of the blood-brain barrier (μBBB).Lab Chip. 2012 Apr 24;12(10):1784-92. doi: 10.1039/c2lc40094d. Epub 2012 Mar 15. Lab Chip. 2012. PMID: 22422217
-
Development of Human in vitro Brain-blood Barrier Model from Induced Pluripotent Stem Cell-derived Endothelial Cells to Predict the in vivo Permeability of Drugs.Neurosci Bull. 2019 Dec;35(6):996-1010. doi: 10.1007/s12264-019-00384-7. Epub 2019 May 11. Neurosci Bull. 2019. PMID: 31079318 Free PMC article.
-
BBB on chip: microfluidic platform to mechanically and biochemically modulate blood-brain barrier function.Biomed Microdevices. 2013 Feb;15(1):145-50. doi: 10.1007/s10544-012-9699-7. Biomed Microdevices. 2013. PMID: 22955726
-
Human iPSC-Derived Blood-Brain Barrier Models: Valuable Tools for Preclinical Drug Discovery and Development?Curr Protoc Stem Cell Biol. 2020 Dec;55(1):e122. doi: 10.1002/cpsc.122. Curr Protoc Stem Cell Biol. 2020. PMID: 32956578 Review.
-
Microfluidic organ-on-chip technology for blood-brain barrier research.Tissue Barriers. 2016 Jan 28;4(1):e1142493. doi: 10.1080/21688370.2016.1142493. eCollection 2016 Jan-Mar. Tissue Barriers. 2016. PMID: 27141422 Free PMC article. Review.
Cited by
-
Host-microbe interactions at the blood-brain barrier through the lens of induced pluripotent stem cell-derived brain-like endothelial cells.mBio. 2024 Feb 14;15(2):e0286223. doi: 10.1128/mbio.02862-23. Epub 2024 Jan 9. mBio. 2024. PMID: 38193670 Free PMC article. Review.
-
Are In Vitro Human Blood-Brain-Tumor-Barriers Suitable Replacements for In Vivo Models of Brain Permeability for Novel Therapeutics?Cancers (Basel). 2021 Feb 25;13(5):955. doi: 10.3390/cancers13050955. Cancers (Basel). 2021. PMID: 33668807 Free PMC article. Review.
-
Dissecting and rebuilding the glioblastoma microenvironment with engineered materials.Nat Rev Mater. 2019 Oct;4(10):651-668. doi: 10.1038/s41578-019-0135-y. Epub 2019 Aug 16. Nat Rev Mater. 2019. PMID: 32647587 Free PMC article.
-
Revolutionizing Drug Discovery: The Impact of Distinct Designs and Biosensor Integration in Microfluidics-Based Organ-on-a-Chip Technology.Biosensors (Basel). 2024 Sep 3;14(9):425. doi: 10.3390/bios14090425. Biosensors (Basel). 2024. PMID: 39329800 Free PMC article. Review.
-
A human in vitro platform for the evaluation of pharmacology strategies in cardiac ischemia.APL Bioeng. 2019 Aug 13;3(3):036103. doi: 10.1063/1.5089237. eCollection 2019 Sep. APL Bioeng. 2019. PMID: 31431939 Free PMC article.
References
-
- Abaci HE, Gledhill K, Guo Z, Christiano AM, Shuler ML. 2015. Pumpless microfluidic platform for drug testing on human skin equivalents. Lab Chip 15:882–888. http://xlink.rsc.org/?DOI=C4LC00999A. - PMC - PubMed
-
- Abaci H, Shuler M. 2015. Human-on-a-Chip Design Strategies and Principles for Physiologically Based Pharmocokinetics/Pharmacodynamics Modeling. Integr. Biol. 7:383–391. http://pubs.rsc.org/en/Content/ArticleLanding/2015/IB/C4IB00292J. - PMC - PubMed
-
- Achyuta AKH, Conway AJ, Crouse RB, Bannister EC, Lee RN, Katnik CP, Behensky A a.,Cuevas J, Sundaram SS. 2013. A modular approach to create a neurovascularunit-on-a-chip. Lab Chip 212:542–553. - PubMed
-
- Avdeef A 2012. Absorption and Drug Development: Solubility, Permeability, and Charge State. Absorpt. Drug Dev. Solubility, Permeability, Charg. State.
Publication types
MeSH terms
Grants and funding
LinkOut - more resources
Full Text Sources
Other Literature Sources