Molecular basis of the remarkable species selectivity of an insecticidal sodium channel toxin from the African spider Augacephalus ezendami
- PMID: 27383378
- PMCID: PMC4935840
- DOI: 10.1038/srep29538
Molecular basis of the remarkable species selectivity of an insecticidal sodium channel toxin from the African spider Augacephalus ezendami
Abstract
The inexorable decline in the armament of registered chemical insecticides has stimulated research into environmentally-friendly alternatives. Insecticidal spider-venom peptides are promising candidates for bioinsecticide development but it is challenging to find peptides that are specific for targeted pests. In the present study, we isolated an insecticidal peptide (Ae1a) from venom of the African spider Augacephalus ezendami (family Theraphosidae). Injection of Ae1a into sheep blowflies (Lucilia cuprina) induced rapid but reversible paralysis. In striking contrast, Ae1a was lethal to closely related fruit flies (Drosophila melanogaster) but induced no adverse effects in the recalcitrant lepidopteran pest Helicoverpa armigera. Electrophysiological experiments revealed that Ae1a potently inhibits the voltage-gated sodium channel BgNaV1 from the German cockroach Blattella germanica by shifting the threshold for channel activation to more depolarized potentials. In contrast, Ae1a failed to significantly affect sodium currents in dorsal unpaired median neurons from the American cockroach Periplaneta americana. We show that Ae1a interacts with the domain II voltage sensor and that sensitivity to the toxin is conferred by natural sequence variations in the S1-S2 loop of domain II. The phyletic specificity of Ae1a provides crucial information for development of sodium channel insecticides that target key insect pests without harming beneficial species.
Figures
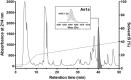
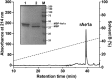
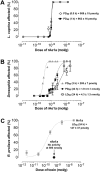
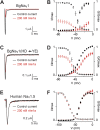
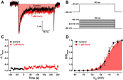
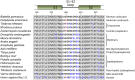
Similar articles
-
Insect-Active Toxins with Promiscuous Pharmacology from the African Theraphosid Spider Monocentropus balfouri.Toxins (Basel). 2017 May 5;9(5):155. doi: 10.3390/toxins9050155. Toxins (Basel). 2017. PMID: 28475112 Free PMC article.
-
The insecticidal neurotoxin Aps III is an atypical knottin peptide that potently blocks insect voltage-gated sodium channels.Biochem Pharmacol. 2013 May 15;85(10):1542-54. doi: 10.1016/j.bcp.2013.02.030. Epub 2013 Mar 6. Biochem Pharmacol. 2013. PMID: 23473802 Free PMC article.
-
The insecticidal spider toxin SFI1 is a knottin peptide that blocks the pore of insect voltage-gated sodium channels via a large β-hairpin loop.FEBS J. 2015 Mar;282(5):904-20. doi: 10.1111/febs.13189. Epub 2015 Jan 23. FEBS J. 2015. PMID: 25559770
-
Spider and scorpion knottins targeting voltage-gated sodium ion channels in pain signaling.Biochem Pharmacol. 2024 Sep;227:116465. doi: 10.1016/j.bcp.2024.116465. Epub 2024 Aug 3. Biochem Pharmacol. 2024. PMID: 39102991 Review.
-
A complicated complex: Ion channels, voltage sensing, cell membranes and peptide inhibitors.Neurosci Lett. 2018 Jul 13;679:35-47. doi: 10.1016/j.neulet.2018.04.030. Epub 2018 Apr 21. Neurosci Lett. 2018. PMID: 29684532 Review.
Cited by
-
Isolation of two insecticidal toxins from venom of the Australian theraphosid spider Coremiocnemis tropix.Toxicon. 2016 Dec 1;123:62-70. doi: 10.1016/j.toxicon.2016.10.013. Epub 2016 Oct 26. Toxicon. 2016. PMID: 27793656 Free PMC article.
-
Purification and Characterization of a Novel Insecticidal Toxin, μ-sparatoxin-Hv2, from the Venom of the Spider Heteropoda venatoria.Toxins (Basel). 2018 Jun 7;10(6):233. doi: 10.3390/toxins10060233. Toxins (Basel). 2018. PMID: 29880771 Free PMC article.
-
Spider-Venom Peptides: Structure, Bioactivity, Strategy, and Research Applications.Molecules. 2023 Dec 20;29(1):35. doi: 10.3390/molecules29010035. Molecules. 2023. PMID: 38202621 Free PMC article. Review.
-
Characterization of Sodium Channel Peptides Obtained from the Venom of the Scorpion Centruroides bonito.Toxins (Basel). 2024 Mar 1;16(3):125. doi: 10.3390/toxins16030125. Toxins (Basel). 2024. PMID: 38535792 Free PMC article.
-
A neurotoxin that specifically targets Anopheles mosquitoes.Nat Commun. 2019 Jun 28;10(1):2869. doi: 10.1038/s41467-019-10732-w. Nat Commun. 2019. PMID: 31253776 Free PMC article.
References
-
- Kuhn-Nentwig L., Stöcklin R. & Nentwig W. In Spider Physiology and Behaviour—Physiology (ed. Casas J. ) 1–86 (Elsevier, 2011).
-
- King G. F. & Hardy M. C. Spider-venom peptides: structure, pharmacology, and potential for control of insect pests. Annu. Rev. Entomol. 58, 475–496 (2013). - PubMed
-
- Escoubas P., Sollod B. & King G. F. Venom landscapes: mining the complexity of spider venoms via a combined cDNA and mass spectrometric approach. Toxicon 47, 650–663 (2006). - PubMed
-
- Maggio F., Sollod B. L., Tedford H. W., Herzig V. & King G. F. In Insect Pharmacology: Channels, Receptors, Toxins and Enzymes (eds Gilbert L. I. & Gill S. S. ) 101–123 (Academic Press, Oxford, 2010).
Publication types
MeSH terms
Substances
LinkOut - more resources
Full Text Sources
Other Literature Sources
Miscellaneous