Human TRPA1 is a heat sensor displaying intrinsic U-shaped thermosensitivity
- PMID: 27349477
- PMCID: PMC4923899
- DOI: 10.1038/srep28763
Human TRPA1 is a heat sensor displaying intrinsic U-shaped thermosensitivity
Abstract
Thermosensitive Transient Receptor Potential (TRP) channels are believed to respond to either cold or heat. In the case of TRP subtype A1 (TRPA1), there seems to be a species-dependent divergence in temperature sensation as non-mammalian TRPA1 is heat-sensitive whereas mammalian TRPA1 is sensitive to cold. It has been speculated but never experimentally proven that TRPA1 and other temperature-sensitive ion channels have the inherent capability of responding to both cold and heat. Here we show that redox modification and ligands affect human TRPA1 (hTRPA1) cold and heat sensing properties in lipid bilayer and whole-cell patch-clamp recordings as well as heat-evoked TRPA1-dependent calcitonin gene-related peptide (CGRP) release from mouse trachea. Studies of purified hTRPA1 intrinsic tryptophan fluorescence, in the absence of lipid bilayer, consolidate hTRPA1 as an intrinsic bidirectional thermosensor that is modified by the redox state and ligands. Thus, the heat sensing property of TRPA1 is conserved in mammalians, in which TRPA1 may contribute to sensing warmth and uncomfortable heat in addition to noxious cold.
Figures
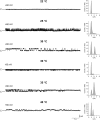
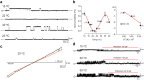
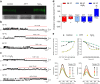
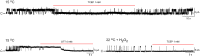
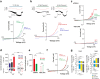
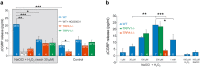
Similar articles
-
The human TRPA1 intrinsic cold and heat sensitivity involves separate channel structures beyond the N-ARD domain.Nat Commun. 2022 Oct 17;13(1):6113. doi: 10.1038/s41467-022-33876-8. Nat Commun. 2022. PMID: 36253390 Free PMC article.
-
Human and Mouse TRPA1 Are Heat and Cold Sensors Differentially Tuned by Voltage.Cells. 2019 Dec 24;9(1):57. doi: 10.3390/cells9010057. Cells. 2019. PMID: 31878344 Free PMC article.
-
TRPA1 expression levels and excitability brake by KV channels influence cold sensitivity of TRPA1-expressing neurons.Neuroscience. 2017 Jun 14;353:76-86. doi: 10.1016/j.neuroscience.2017.04.001. Epub 2017 Apr 10. Neuroscience. 2017. PMID: 28408328 Free PMC article.
-
TRP channels in oxygen physiology: distinctive functional properties and roles of TRPA1 in O2 sensing.Proc Jpn Acad Ser B Phys Biol Sci. 2017;93(7):464-482. doi: 10.2183/pjab.93.028. Proc Jpn Acad Ser B Phys Biol Sci. 2017. PMID: 28769017 Free PMC article. Review.
-
Transient receptor potential ankyrin 1 channel: An evolutionarily tuned thermosensor.Physiol Res. 2021 Jul 12;70(3):363-381. doi: 10.33549/physiolres.934697. Epub 2021 May 12. Physiol Res. 2021. PMID: 33982589 Free PMC article. Review.
Cited by
-
Human surrogate models of central sensitization: A critical review and practical guide.Eur J Pain. 2021 Aug;25(7):1389-1428. doi: 10.1002/ejp.1768. Epub 2021 May 8. Eur J Pain. 2021. PMID: 33759294 Free PMC article. Review.
-
TRPA1 Antagonists for Pain Relief.Pharmaceuticals (Basel). 2018 Nov 1;11(4):117. doi: 10.3390/ph11040117. Pharmaceuticals (Basel). 2018. PMID: 30388732 Free PMC article. Review.
-
Drosophila menthol sensitivity and the Precambrian origins of transient receptor potential-dependent chemosensation.Philos Trans R Soc Lond B Biol Sci. 2019 Nov 11;374(1785):20190369. doi: 10.1098/rstb.2019.0369. Epub 2019 Sep 23. Philos Trans R Soc Lond B Biol Sci. 2019. PMID: 31544603 Free PMC article.
-
TRPA5 encodes a thermosensitive ankyrin ion channel receptor in a triatomine insect.iScience. 2024 Mar 20;27(4):109541. doi: 10.1016/j.isci.2024.109541. eCollection 2024 Apr 19. iScience. 2024. PMID: 38577108 Free PMC article.
-
Activation of Drosophila melanogaster TRPA1 Isoforms by Citronellal and Menthol.Int J Mol Sci. 2021 Oct 12;22(20):10997. doi: 10.3390/ijms222010997. Int J Mol Sci. 2021. PMID: 34681657 Free PMC article.
References
-
- Story G. M. et al.. ANKTM1, a TRP-like channel expressed in nociceptive neurons, is activated by cold temperatures. Cell 112, 819–829 (2003). - PubMed
-
- Zygmunt P. M. & Högestätt E. D. Trpa1. Handb Exp Pharmacol 222, 583–630 (2014). - PubMed
-
- Albin K. C., Carstens M. I. & Carstens E. Modulation of oral heat and cold pain by irritant chemicals. Chem Senses 33, 3–15 (2008). - PubMed
-
- Koltzenburg M., Lundberg L. E. & Torebjork H. E. Dynamic and static components of mechanical hyperalgesia in human hairy skin. Pain 51, 207–219 (1992). - PubMed
Publication types
MeSH terms
Substances
LinkOut - more resources
Full Text Sources
Other Literature Sources
Research Materials