Rebuilding the Damaged Heart: Mesenchymal Stem Cells, Cell-Based Therapy, and Engineered Heart Tissue
- PMID: 27335447
- PMCID: PMC6345247
- DOI: 10.1152/physrev.00019.2015
Rebuilding the Damaged Heart: Mesenchymal Stem Cells, Cell-Based Therapy, and Engineered Heart Tissue
Abstract
Mesenchymal stem cells (MSCs) are broadly distributed cells that retain postnatal capacity for self-renewal and multilineage differentiation. MSCs evade immune detection, secrete an array of anti-inflammatory and anti-fibrotic mediators, and very importantly activate resident precursors. These properties form the basis for the strategy of clinical application of cell-based therapeutics for inflammatory and fibrotic conditions. In cardiovascular medicine, administration of autologous or allogeneic MSCs in patients with ischemic and nonischemic cardiomyopathy holds significant promise. Numerous preclinical studies of ischemic and nonischemic cardiomyopathy employing MSC-based therapy have demonstrated that the properties of reducing fibrosis, stimulating angiogenesis, and cardiomyogenesis have led to improvements in the structure and function of remodeled ventricles. Further attempts have been made to augment MSCs' effects through genetic modification and cell preconditioning. Progression of MSC therapy to early clinical trials has supported their role in improving cardiac structure and function, functional capacity, and patient quality of life. Emerging data have supported larger clinical trials that have been either completed or are currently underway. Mechanistically, MSC therapy is thought to benefit the heart by stimulating innate anti-fibrotic and regenerative responses. The mechanisms of action involve paracrine signaling, cell-cell interactions, and fusion with resident cells. Trans-differentiation of MSCs to bona fide cardiomyocytes and coronary vessels is also thought to occur, although at a nonphysiological level. Recently, MSC-based tissue engineering for cardiovascular disease has been examined with quite encouraging results. This review discusses MSCs from their basic biological characteristics to their role as a promising therapeutic strategy for clinical cardiovascular disease.
Copyright © 2016 the American Physiological Society.
Figures
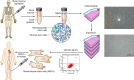
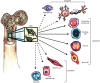
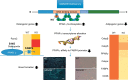
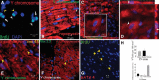
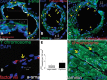
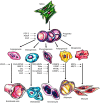
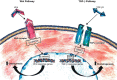
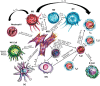
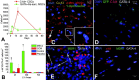
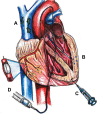
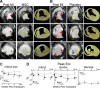
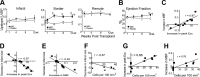
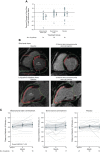
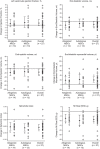
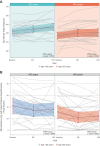
Similar articles
-
Optimization of the cardiovascular therapeutic properties of mesenchymal stromal/stem cells-taking the next step.Stem Cell Rev Rep. 2013 Jun;9(3):281-302. doi: 10.1007/s12015-012-9366-7. Stem Cell Rev Rep. 2013. PMID: 22529015 Review.
-
Autologous mesenchymal stem cells produce concordant improvements in regional function, tissue perfusion, and fibrotic burden when administered to patients undergoing coronary artery bypass grafting: The Prospective Randomized Study of Mesenchymal Stem Cell Therapy in Patients Undergoing Cardiac Surgery (PROMETHEUS) trial.Circ Res. 2014 Apr 11;114(8):1302-10. doi: 10.1161/CIRCRESAHA.114.303180. Epub 2014 Feb 24. Circ Res. 2014. PMID: 24565698 Free PMC article. Clinical Trial.
-
Application and Progress of Combined Mesenchymal Stem Cell Transplantation in the Treatment of Ischemic Cardiomyopathy.Biomed Res Int. 2015;2015:568502. doi: 10.1155/2015/568502. Epub 2015 Jul 29. Biomed Res Int. 2015. PMID: 26295041 Free PMC article. Review.
-
Surfing the clinical trials of mesenchymal stem cell therapy in ischemic cardiomyopathy.Stem Cell Res Ther. 2021 Jun 23;12(1):361. doi: 10.1186/s13287-021-02443-1. Stem Cell Res Ther. 2021. PMID: 34162424 Free PMC article. Review.
-
Pre-Conditioning Methods and Novel Approaches with Mesenchymal Stem Cells Therapy in Cardiovascular Disease.Cells. 2022 May 12;11(10):1620. doi: 10.3390/cells11101620. Cells. 2022. PMID: 35626657 Free PMC article. Review.
Cited by
-
Mesenchymal stem cells as future treatment for cardiovascular regeneration and its challenges.Ann Transl Med. 2024 Aug 1;12(4):73. doi: 10.21037/atm-23-1936. Epub 2023 Dec 29. Ann Transl Med. 2024. PMID: 39118948 Free PMC article. Review.
-
Enhancement Strategies for Cardiac Regenerative Cell Therapy: Focus on Adult Stem Cells.Circ Res. 2018 Jul 6;123(2):177-187. doi: 10.1161/CIRCRESAHA.118.311207. Circ Res. 2018. PMID: 29976686 Free PMC article. Review.
-
The Optimal Intervention Time of Bone Marrow Mesenchymal Stem Cells in Ameliorating Cardiac Fibrosis Induced by Viral Myocarditis: A Randomized Controlled Trial in Mice.Stem Cells Int. 2017;2017:3258035. doi: 10.1155/2017/3258035. Epub 2017 Dec 5. Stem Cells Int. 2017. PMID: 29362568 Free PMC article.
-
Regenerative Medicine for the Treatment of Ischemic Heart Disease; Status and Future Perspectives.Front Cell Dev Biol. 2021 Sep 10;9:704903. doi: 10.3389/fcell.2021.704903. eCollection 2021. Front Cell Dev Biol. 2021. PMID: 34568321 Free PMC article. Review.
-
Randomized Comparison of Allogeneic Versus Autologous Mesenchymal Stem Cells for Nonischemic Dilated Cardiomyopathy: POSEIDON-DCM Trial.J Am Coll Cardiol. 2017 Feb 7;69(5):526-537. doi: 10.1016/j.jacc.2016.11.009. Epub 2016 Nov 14. J Am Coll Cardiol. 2017. PMID: 27856208 Free PMC article. Clinical Trial.
References
-
- Stem the tide. Nature : 163–164, 2015. - PubMed
-
- Abdel-Latif A, Bolli R, Tleyjeh IM, Montori VM, Perin EC, Hornung CA, Zuba-Surma EK, Al-Mallah M, Dawn B. Adult bone marrow-derived cells for cardiac repair: a systematic review and meta-analysis. Arch Int Med : 989–997, 2007. - PubMed
-
- Aggarwal S, Pittenger MF. Human mesenchymal stem cells modulate allogeneic immune cell responses. Blood : 1815–1822, 2005. - PubMed
-
- Ahmad T, Mukherjee S, Pattnaik B, Kumar M, Singh S, Kumar M, Rehman R, Tiwari BK, Jha KA, Barhanpurkar AP, Wani MR, Roy SS, Mabalirajan U, Ghosh B, Agrawal A. Miro1 regulates intercellular mitochondrial transport and enhances mesenchymal stem cell rescue efficacy. EMBO J : 994–1010, 2014. - PMC - PubMed
Publication types
MeSH terms
Grants and funding
LinkOut - more resources
Full Text Sources
Other Literature Sources
Medical