Gap junctional signaling in pattern regulation: Physiological network connectivity instructs growth and form
- PMID: 27265625
- PMCID: PMC10478170
- DOI: 10.1002/dneu.22405
Gap junctional signaling in pattern regulation: Physiological network connectivity instructs growth and form
Abstract
Gap junctions (GJs) are aqueous channels that allow cells to communicate via physiological signals directly. The role of gap junctional connectivity in determining single-cell functions has long been recognized. However, GJs have another important role: the regulation of large-scale anatomical pattern. GJs are not only versatile computational elements that allow cells to control which small molecule signals they receive and emit, but also establish connectivity patterns within large groups of cells. By dynamically regulating the topology of bioelectric networks in vivo, GJs underlie the ability of many tissues to implement complex morphogenesis. Here, a review of recent data on patterning roles of GJs in growth of the zebrafish fin, the establishment of left-right patterning, the developmental dysregulation known as cancer, and the control of large-scale head-tail polarity, and head shape in planarian regeneration has been reported. A perspective in which GJs are not only molecular features functioning in single cells, but also enable global neural-like dynamics in non-neural somatic tissues has been proposed. This view suggests a rich program of future work which capitalizes on the rapid advances in the biophysics of GJs to exploit GJ-mediated global dynamics for applications in birth defects, regenerative medicine, and morphogenetic bioengineering. © 2016 Wiley Periodicals, Inc. Develop Neurobiol 77: 643-673, 2017.
Keywords: bioelectric; gap junctions; morphogenesis; networks; patterning.
© 2016 Wiley Periodicals, Inc.
Conflict of interest statement
The authors declare no conflicts or competing interests.
Figures
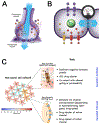
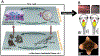
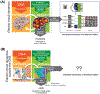
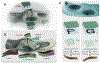
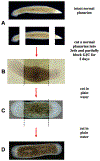
Similar articles
-
Morphology changes induced by intercellular gap junction blocking: A reaction-diffusion mechanism.Biosystems. 2021 Nov;209:104511. doi: 10.1016/j.biosystems.2021.104511. Epub 2021 Aug 17. Biosystems. 2021. PMID: 34411690
-
Endogenous Bioelectric Signaling Networks: Exploiting Voltage Gradients for Control of Growth and Form.Annu Rev Biomed Eng. 2017 Jun 21;19:353-387. doi: 10.1146/annurev-bioeng-071114-040647. Annu Rev Biomed Eng. 2017. PMID: 28633567 Free PMC article. Review.
-
The contribution of electrical synapses to field potential oscillations in the hippocampal formation.Front Neural Circuits. 2014 Apr 3;8:32. doi: 10.3389/fncir.2014.00032. eCollection 2014. Front Neural Circuits. 2014. PMID: 24772068 Free PMC article. Review.
-
Endogenous gradients of resting potential instructively pattern embryonic neural tissue via Notch signaling and regulation of proliferation.J Neurosci. 2015 Mar 11;35(10):4366-85. doi: 10.1523/JNEUROSCI.1877-14.2015. J Neurosci. 2015. PMID: 25762681 Free PMC article.
-
Bioelectrical model of head-tail patterning based on cell ion channels and intercellular gap junctions.Bioelectrochemistry. 2020 Apr;132:107410. doi: 10.1016/j.bioelechem.2019.107410. Epub 2019 Nov 29. Bioelectrochemistry. 2020. PMID: 31821903
Cited by
-
Modeling somatic computation with non-neural bioelectric networks.Sci Rep. 2019 Dec 9;9(1):18612. doi: 10.1038/s41598-019-54859-8. Sci Rep. 2019. PMID: 31819119 Free PMC article.
-
The Yin and Yang of Breast Cancer: Ion Channels as Determinants of Left-Right Functional Differences.Int J Mol Sci. 2023 Jul 5;24(13):11121. doi: 10.3390/ijms241311121. Int J Mol Sci. 2023. PMID: 37446299 Free PMC article.
-
HCN2 Channel-Induced Rescue of Brain Teratogenesis via Local and Long-Range Bioelectric Repair.Front Cell Neurosci. 2020 May 26;14:136. doi: 10.3389/fncel.2020.00136. eCollection 2020. Front Cell Neurosci. 2020. PMID: 32528251 Free PMC article.
-
Optical Imaging of Electrical and Mechanical Couplings between Cells.ACS Sens. 2021 Feb 26;6(2):508-512. doi: 10.1021/acssensors.0c02058. Epub 2020 Dec 22. ACS Sens. 2021. PMID: 33351601 Free PMC article.
-
Connexin 43 gap junctional intercellular communication inhibits evx1 expression and joint formation in regenerating fins.Development. 2020 Jul 3;147(13):dev190512. doi: 10.1242/dev.190512. Development. 2020. PMID: 32541014 Free PMC article.
References
Publication types
MeSH terms
Grants and funding
LinkOut - more resources
Full Text Sources
Other Literature Sources
Miscellaneous