Ribosome recycling defects modify the balance between the synthesis and assembly of specific subunits of the oxidative phosphorylation complexes in yeast mitochondria
- PMID: 27257059
- PMCID: PMC4937339
- DOI: 10.1093/nar/gkw490
Ribosome recycling defects modify the balance between the synthesis and assembly of specific subunits of the oxidative phosphorylation complexes in yeast mitochondria
Abstract
Mitochondria have their own translation machinery that produces key subunits of the OXPHOS complexes. This machinery relies on the coordinated action of nuclear-encoded factors of bacterial origin that are well conserved between humans and yeast. In humans, mutations in these factors can cause diseases; in yeast, mutations abolishing mitochondrial translation destabilize the mitochondrial DNA. We show that when the mitochondrial genome contains no introns, the loss of the yeast factors Mif3 and Rrf1 involved in ribosome recycling neither blocks translation nor destabilizes mitochondrial DNA. Rather, the absence of these factors increases the synthesis of the mitochondrially-encoded subunits Cox1, Cytb and Atp9, while strongly impairing the assembly of OXPHOS complexes IV and V. We further show that in the absence of Rrf1, the COX1 specific translation activator Mss51 accumulates in low molecular weight forms, thought to be the source of the translationally-active form, explaining the increased synthesis of Cox1. We propose that Rrf1 takes part in the coordination between translation and OXPHOS assembly in yeast mitochondria. These interactions between general and specific translation factors might reveal an evolutionary adaptation of the bacterial translation machinery to the set of integral membrane proteins that are translated within mitochondria.
© The Author(s) 2016. Published by Oxford University Press on behalf of Nucleic Acids Research.
Figures
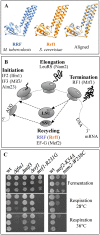
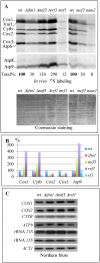
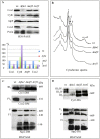
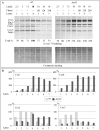
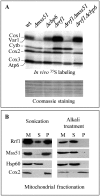
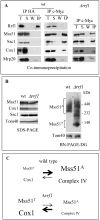
Similar articles
-
Translational activators and mitoribosomal isoforms cooperate to mediate mRNA-specific translation in Schizosaccharomyces pombe mitochondria.Nucleic Acids Res. 2021 Nov 8;49(19):11145-11166. doi: 10.1093/nar/gkab789. Nucleic Acids Res. 2021. PMID: 34634819 Free PMC article.
-
Atco, a yeast mitochondrial complex of Atp9 and Cox6, is an assembly intermediate of the ATP synthase.PLoS One. 2020 May 15;15(5):e0233177. doi: 10.1371/journal.pone.0233177. eCollection 2020. PLoS One. 2020. PMID: 32413073 Free PMC article.
-
Oxa1 directly interacts with Atp9 and mediates its assembly into the mitochondrial F1Fo-ATP synthase complex.Mol Biol Cell. 2007 May;18(5):1897-908. doi: 10.1091/mbc.e06-10-0925. Epub 2007 Mar 7. Mol Biol Cell. 2007. PMID: 17344477 Free PMC article.
-
Activation of Yeast Mitochondrial Translation: Who Is in Charge?Biochemistry (Mosc). 2018 Feb;83(2):87-97. doi: 10.1134/S0006297918020013. Biochemistry (Mosc). 2018. PMID: 29618295 Review.
-
Mechanisms of mitochondrial translational regulation.IUBMB Life. 2013 May;65(5):397-408. doi: 10.1002/iub.1156. Epub 2013 Apr 3. IUBMB Life. 2013. PMID: 23554047 Review.
Cited by
-
The ribosome receptors Mrx15 and Mba1 jointly organize cotranslational insertion and protein biogenesis in mitochondria.Mol Biol Cell. 2018 Oct 1;29(20):2386-2396. doi: 10.1091/mbc.E18-04-0227. Epub 2018 Aug 9. Mol Biol Cell. 2018. PMID: 30091672 Free PMC article.
-
Mitochondrial Protein Translation: Emerging Roles and Clinical Significance in Disease.Front Cell Dev Biol. 2021 Jul 1;9:675465. doi: 10.3389/fcell.2021.675465. eCollection 2021. Front Cell Dev Biol. 2021. PMID: 34277617 Free PMC article. Review.
-
MrpL35, a mitospecific component of mitoribosomes, plays a key role in cytochrome c oxidase assembly.Mol Biol Cell. 2017 Nov 15;28(24):3489-3499. doi: 10.1091/mbc.E17-04-0239. Epub 2017 Sep 20. Mol Biol Cell. 2017. PMID: 28931599 Free PMC article.
-
Mechanisms of ribosome recycling in bacteria and mitochondria: a structural perspective.RNA Biol. 2022;19(1):662-677. doi: 10.1080/15476286.2022.2067712. Epub 2021 Dec 31. RNA Biol. 2022. PMID: 35485608 Free PMC article. Review.
-
Yeast Mitochondrial Translation Initiation Factor 3 Interacts with Pet111p to Promote COX2 mRNA Translation.Int J Mol Sci. 2020 May 12;21(10):3414. doi: 10.3390/ijms21103414. Int J Mol Sci. 2020. PMID: 32408541 Free PMC article.
References
-
- Hirokawa G., Demeshkina N., Iwakura N., Kaji H., Kaji A. The ribosome-recycling step: consensus or controversy? Trends Biochem. Sci. 2006;31:143–149. - PubMed
-
- Seshadri A., Varshney U. Mechanism of recycling of post-termination ribosomal complexes in eubacteria: a new role of initiation factor 3. J. Biosci. 2006;31:281–289. - PubMed
MeSH terms
Substances
LinkOut - more resources
Full Text Sources
Other Literature Sources
Molecular Biology Databases