Mechanism of impaired microtubule-dependent peroxisome trafficking and oxidative stress in SPAST-mutated cells from patients with Hereditary Spastic Paraplegia
- PMID: 27229699
- PMCID: PMC4882512
- DOI: 10.1038/srep27004
Mechanism of impaired microtubule-dependent peroxisome trafficking and oxidative stress in SPAST-mutated cells from patients with Hereditary Spastic Paraplegia
Abstract
Hereditary spastic paraplegia (HSP) is an inherited neurological condition that leads to progressive spasticity and gait abnormalities. Adult-onset HSP is most commonly caused by mutations in SPAST, which encodes spastin a microtubule severing protein. In olfactory stem cell lines derived from patients carrying different SPAST mutations, we investigated microtubule-dependent peroxisome movement with time-lapse imaging and automated image analysis. The average speed of peroxisomes in patient-cells was slower, with fewer fast moving peroxisomes than in cells from healthy controls. This was not because of impairment of peroxisome-microtubule interactions because the time-dependent saltatory dynamics of movement of individual peroxisomes was unaffected in patient-cells. Our observations indicate that average peroxisome speeds are less in patient-cells because of the lower probability of individual peroxisome interactions with the reduced numbers of stable microtubules: peroxisome speeds in patient cells are restored by epothilone D, a tubulin-binding drug that increases the number of stable microtubules to control levels. Patient-cells were under increased oxidative stress and were more sensitive than control-cells to hydrogen peroxide, which is primarily metabolised by peroxisomal catalase. Epothilone D also ameliorated patient-cell sensitivity to hydrogen-peroxide. Our findings suggest a mechanism for neurodegeneration whereby SPAST mutations indirectly lead to impaired peroxisome transport and oxidative stress.
Figures
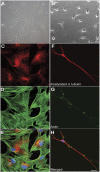
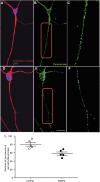
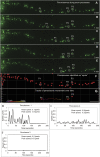
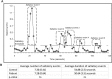
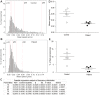
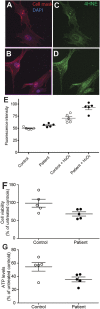
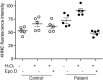
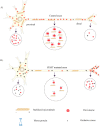
Similar articles
-
Oxidative Stress-Induced Axon Fragmentation Is a Consequence of Reduced Axonal Transport in Hereditary Spastic Paraplegia SPAST Patient Neurons.Front Neurosci. 2020 May 7;14:401. doi: 10.3389/fnins.2020.00401. eCollection 2020. Front Neurosci. 2020. PMID: 32457567 Free PMC article.
-
A patient-derived stem cell model of hereditary spastic paraplegia with SPAST mutations.Dis Model Mech. 2013 Mar;6(2):489-502. doi: 10.1242/dmm.010884. Epub 2012 Dec 20. Dis Model Mech. 2013. PMID: 23264559 Free PMC article.
-
Hereditary spastic paraplegia SPG4: what is known and not known about the disease.Brain. 2015 Sep;138(Pt 9):2471-84. doi: 10.1093/brain/awv178. Epub 2015 Jun 20. Brain. 2015. PMID: 26094131 Free PMC article. Review.
-
MiR-33a is a therapeutic target in SPG4-related hereditary spastic paraplegia human neurons.Clin Sci (Lond). 2019 Feb 22;133(4):583-595. doi: 10.1042/CS20180980. Print 2019 Feb 28. Clin Sci (Lond). 2019. PMID: 30777884
-
Tau missorting and spastin-induced microtubule disruption in neurodegeneration: Alzheimer Disease and Hereditary Spastic Paraplegia.Mol Neurodegener. 2015 Dec 21;10:68. doi: 10.1186/s13024-015-0064-1. Mol Neurodegener. 2015. PMID: 26691836 Free PMC article. Review.
Cited by
-
Patient-Derived Stem Cell Models in SPAST HSP: Disease Modelling and Drug Discovery.Brain Sci. 2018 Jul 31;8(8):142. doi: 10.3390/brainsci8080142. Brain Sci. 2018. PMID: 30065201 Free PMC article. Review.
-
Reduced acetylated α-tubulin in SPAST hereditary spastic paraplegia patient PBMCs.Front Neurosci. 2023 Mar 28;17:1073516. doi: 10.3389/fnins.2023.1073516. eCollection 2023. Front Neurosci. 2023. PMID: 37144097 Free PMC article.
-
Mutant spastin proteins promote deficits in axonal transport through an isoform-specific mechanism involving casein kinase 2 activation.Hum Mol Genet. 2017 Jun 15;26(12):2321-2334. doi: 10.1093/hmg/ddx125. Hum Mol Genet. 2017. PMID: 28398512 Free PMC article.
-
Serum neurofilament light chain is increased in hereditary spastic paraplegias.Ann Clin Transl Neurol. 2018 May 21;5(7):876-882. doi: 10.1002/acn3.583. eCollection 2018 Jul. Ann Clin Transl Neurol. 2018. PMID: 30009206 Free PMC article.
-
Oxidative Stress-Induced Axon Fragmentation Is a Consequence of Reduced Axonal Transport in Hereditary Spastic Paraplegia SPAST Patient Neurons.Front Neurosci. 2020 May 7;14:401. doi: 10.3389/fnins.2020.00401. eCollection 2020. Front Neurosci. 2020. PMID: 32457567 Free PMC article.
References
Publication types
MeSH terms
Substances
LinkOut - more resources
Full Text Sources
Other Literature Sources