Role of Conserved Disulfide Bridges and Aromatic Residues in Extracellular Loop 2 of Chemokine Receptor CCR8 for Chemokine and Small Molecule Binding
- PMID: 27226537
- PMCID: PMC4965569
- DOI: 10.1074/jbc.M115.706747
Role of Conserved Disulfide Bridges and Aromatic Residues in Extracellular Loop 2 of Chemokine Receptor CCR8 for Chemokine and Small Molecule Binding
Abstract
Chemokine receptors play important roles in the immune system and are linked to several human diseases. The initial contact of chemokines with their receptors depends on highly specified extracellular receptor features. Here we investigate the importance of conserved extracellular disulfide bridges and aromatic residues in extracellular loop 2 (ECL-2) for ligand binding and activation in the chemokine receptor CCR8. We used inositol 1,4,5-trisphosphate accumulation and radioligand binding experiments to determine the impact of receptor mutagenesis on both chemokine and small molecule agonist and antagonist binding and action in CCR8. We find that the seven-transmembrane (TM) receptor conserved disulfide bridge (7TM bridge) linking transmembrane helix III (TMIII) and ECL-2 is crucial for chemokine and small molecule action, whereas the chemokine receptor conserved disulfide bridge between the N terminus and TMVII is needed only for chemokines. Furthermore, we find that two distinct aromatic residues in ECL-2, Tyr(184) (Cys + 1) and Tyr(187) (Cys + 4), are crucial for binding of the CC chemokines CCL1 (agonist) and MC148 (antagonist), respectively, but not for small molecule binding. Finally, using in silico modeling, we predict an aromatic cluster of interaction partners for Tyr(187) in TMIV (Phe(171)) and TMV (Trp(194)). We show in vitro that these residues are crucial for the binding and action of MC148, thus supporting their participation in an aromatic cluster with Tyr(187) This aromatic cluster appears to be present in a large number of CC chemokine receptors and thereby could play a more general role to be exploited in future drug development targeting these receptors.
Keywords: CCR8; G protein-coupled receptor (GPCR); chemokine receptor; metal ion-protein interaction; molecular pharmacology; mutagenesis in vitro; protein-protein interaction; receptor structure-function.
© 2016 by The American Society for Biochemistry and Molecular Biology, Inc.
Figures
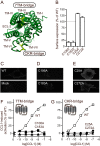
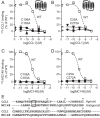
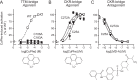
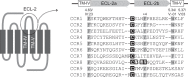
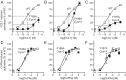
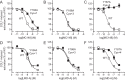
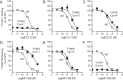
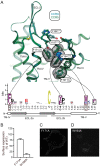
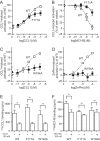
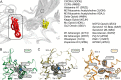
Similar articles
-
Reversed binding of a small molecule ligand in homologous chemokine receptors - differential role of extracellular loop 2.Br J Pharmacol. 2012 May;166(1):258-75. doi: 10.1111/j.1476-5381.2011.01771.x. Br J Pharmacol. 2012. PMID: 22050085 Free PMC article.
-
Molecular requirements for inhibition of the chemokine receptor CCR8--probe-dependent allosteric interactions.Br J Pharmacol. 2012 Nov;167(6):1206-17. doi: 10.1111/j.1476-5381.2012.02076.x. Br J Pharmacol. 2012. PMID: 22708643 Free PMC article.
-
Extracellular disulfide bridges serve different purposes in two homologous chemokine receptors, CCR1 and CCR5.Mol Pharmacol. 2013 Sep;84(3):335-45. doi: 10.1124/mol.113.086702. Epub 2013 Jun 13. Mol Pharmacol. 2013. PMID: 23765404
-
Interaction of chemokines with their receptors--from initial chemokine binding to receptor activating steps.Curr Med Chem. 2014;21(31):3594-614. doi: 10.2174/0929867321666140716093155. Curr Med Chem. 2014. PMID: 25039782 Review.
-
The binding and specificity of chemokine binding proteins, through the lens of experiment and computation.Biomed J. 2022 Jun;45(3):439-453. doi: 10.1016/j.bj.2021.07.004. Epub 2021 Jul 24. Biomed J. 2022. PMID: 34311129 Free PMC article. Review.
Cited by
-
G Protein-Coupled Receptors in the Sweet Spot: Glycosylation and other Post-translational Modifications.ACS Pharmacol Transl Sci. 2020 Mar 17;3(2):237-245. doi: 10.1021/acsptsci.0c00016. eCollection 2020 Apr 10. ACS Pharmacol Transl Sci. 2020. PMID: 32296765 Free PMC article. Review.
-
Biased Signaling of CCL21 and CCL19 Does Not Rely on N-Terminal Differences, but Markedly on the Chemokine Core Domains and Extracellular Loop 2 of CCR7.Front Immunol. 2019 Sep 13;10:2156. doi: 10.3389/fimmu.2019.02156. eCollection 2019. Front Immunol. 2019. PMID: 31572374 Free PMC article.
-
Structural basis of antibody inhibition and chemokine activation of the human CC chemokine receptor 8.Nat Commun. 2023 Dec 1;14(1):7940. doi: 10.1038/s41467-023-43601-8. Nat Commun. 2023. PMID: 38040762 Free PMC article.
-
Distinct Roles of Extracellular Domains in the Epstein-Barr Virus-Encoded BILF1 Receptor for Signaling and Major Histocompatibility Complex Class I Downregulation.mBio. 2019 Jan 15;10(1):e01707-18. doi: 10.1128/mBio.01707-18. mBio. 2019. PMID: 30647152 Free PMC article.
-
Structural and functional analysis of Ccr1l1, a Rodentia-restricted eosinophil-selective chemokine receptor homologue.J Biol Chem. 2021 Jan-Jun;296:100373. doi: 10.1016/j.jbc.2021.100373. Epub 2021 Feb 3. J Biol Chem. 2021. PMID: 33548230 Free PMC article.
References
-
- Gerard C., and Rollins B. J. (2001) Chemokines and disease. Nat. Immunol. 2, 108–115 - PubMed
-
- Rosenkilde M. M., and Schwartz T. W. (2004) The chemokine system: a major regulator of angiogenesis in health and disease. APMIS 112, 481–495 - PubMed
-
- Viola A., and Luster A. D. (2008) Chemokines and their receptors: drug targets in immunity and inflammation. Annu. Rev. Pharmacol. Toxicol. 48, 171–197 - PubMed
-
- Schwarz M. K., and Wells T. N. (2002) New therapeutics that modulate chemokine networks. Nat. Rev. Drug Discov. 1, 347–358 - PubMed
-
- Allen S. J., Crown S. E., and Handel T. M. (2007) Chemokine: receptor structure, interactions, and antagonism. Annu. Rev. Immunol. 25, 787–820 - PubMed
MeSH terms
Substances
Associated data
- Actions
- Actions
- Actions
- Actions
- Actions
LinkOut - more resources
Full Text Sources
Other Literature Sources