Engaging Cervical Spinal Cord Networks to Reenable Volitional Control of Hand Function in Tetraplegic Patients
- PMID: 27198185
- PMCID: PMC5374120
- DOI: 10.1177/1545968316644344
Engaging Cervical Spinal Cord Networks to Reenable Volitional Control of Hand Function in Tetraplegic Patients
Abstract
Background: Paralysis of the upper limbs from spinal cord injury results in an enormous loss of independence in an individual's daily life. Meaningful improvement in hand function is rare after 1 year of tetraparesis. Therapeutic developments that result in even modest gains in hand volitional function will significantly affect the quality of life for patients afflicted with high cervical injury. The ability to neuromodulate the lumbosacral spinal circuitry via epidural stimulation in regaining postural function and volitional control of the legs has been recently shown. A key question is whether a similar neuromodulatory strategy can be used to improve volitional motor control of the upper limbs, that is, performance of motor tasks considered to be less "automatic" than posture and locomotion. In this study, the effects of cervical epidural stimulation on hand function are characterized in subjects with chronic cervical cord injury.
Objective: Herein we show that epidural stimulation can be applied to the chronic injured human cervical spinal cord to promote volitional hand function.
Methods and results: Two subjects implanted with a cervical epidural electrode array demonstrated improved hand strength (approximately 3-fold) and volitional hand control in the presence of epidural stimulation.
Conclusions: The present data are sufficient to suggest that hand motor function in individuals with chronic tetraplegia can be improved with cervical cord neuromodulation and thus should be comprehensively explored as a possible clinical intervention.
Keywords: cervical spinal cord; epidural stimulation; hand function; neuromodulation; spinal cord injury.
© The Author(s) 2016.
Figures
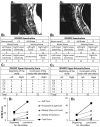
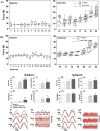
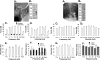
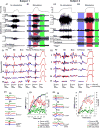
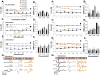
Similar articles
-
Electrical neuromodulation of the cervical spinal cord facilitates forelimb skilled function recovery in spinal cord injured rats.Exp Neurol. 2017 May;291:141-150. doi: 10.1016/j.expneurol.2017.02.006. Epub 2017 Feb 10. Exp Neurol. 2017. PMID: 28192079 Free PMC article.
-
Restoration of hand function with long-term paired associative stimulation after chronic incomplete tetraplegia: a case study.Spinal Cord Ser Cases. 2019 Oct 1;5:81. doi: 10.1038/s41394-019-0225-5. eCollection 2019. Spinal Cord Ser Cases. 2019. PMID: 31632739 Free PMC article.
-
Cortical and Subcortical Effects of Transcutaneous Spinal Cord Stimulation in Humans with Tetraplegia.J Neurosci. 2020 Mar 25;40(13):2633-2643. doi: 10.1523/JNEUROSCI.2374-19.2020. Epub 2020 Jan 29. J Neurosci. 2020. PMID: 31996455 Free PMC article.
-
Emergence of Epidural Electrical Stimulation to Facilitate Sensorimotor Network Functionality After Spinal Cord Injury.Neuromodulation. 2019 Apr;22(3):244-252. doi: 10.1111/ner.12938. Epub 2019 Mar 6. Neuromodulation. 2019. PMID: 30840354 Review.
-
Epidural and transcutaneous spinal electrical stimulation for restoration of movement after incomplete and complete spinal cord injury.Curr Opin Neurol. 2016 Dec;29(6):721-726. doi: 10.1097/WCO.0000000000000382. Curr Opin Neurol. 2016. PMID: 27798422 Review.
Cited by
-
When Spinal Neuromodulation Meets Sensorimotor Rehabilitation: Lessons Learned From Animal Models to Regain Manual Dexterity After a Spinal Cord Injury.Front Rehabil Sci. 2021 Dec 7;2:755963. doi: 10.3389/fresc.2021.755963. eCollection 2021. Front Rehabil Sci. 2021. PMID: 36188826 Free PMC article. Review.
-
Epidural stimulation of the cervical spinal cord for post-stroke upper-limb paresis.Nat Med. 2023 Mar;29(3):689-699. doi: 10.1038/s41591-022-02202-6. Epub 2023 Feb 20. Nat Med. 2023. PMID: 36807682 Free PMC article.
-
Epidural electrical stimulation for spinal cord injury.Neural Regen Res. 2021 Dec;16(12):2367-2375. doi: 10.4103/1673-5374.313017. Neural Regen Res. 2021. PMID: 33907008 Free PMC article. Review.
-
Multi-Site Spinal Cord Transcutaneous Stimulation Facilitates Upper Limb Sensory and Motor Recovery in Severe Cervical Spinal Cord Injury: A Case Study.J Clin Med. 2023 Jun 30;12(13):4416. doi: 10.3390/jcm12134416. J Clin Med. 2023. PMID: 37445450 Free PMC article.
-
Electrical neuromodulation of the cervical spinal cord facilitates forelimb skilled function recovery in spinal cord injured rats.Exp Neurol. 2017 May;291:141-150. doi: 10.1016/j.expneurol.2017.02.006. Epub 2017 Feb 10. Exp Neurol. 2017. PMID: 28192079 Free PMC article.
References
-
- International Campaign for Cures of Spinal Cord Injury Paralysis. 2015 Available at: http://www.campaignforcure.org. - PubMed
Publication types
MeSH terms
Grants and funding
LinkOut - more resources
Full Text Sources
Other Literature Sources