Irc3 is a mitochondrial DNA branch migration enzyme
- PMID: 27194389
- PMCID: PMC4872236
- DOI: 10.1038/srep26414
Irc3 is a mitochondrial DNA branch migration enzyme
Abstract
Integrity of mitochondrial DNA (mtDNA) is essential for cellular energy metabolism. In the budding yeast Saccharomyces cerevisiae, a large number of nuclear genes influence the stability of mitochondrial genome; however, most corresponding gene products act indirectly and the actual molecular mechanisms of mtDNA inheritance remain poorly characterized. Recently, we found that a Superfamily II helicase Irc3 is required for the maintenance of mitochondrial genome integrity. Here we show that Irc3 is a mitochondrial DNA branch migration enzyme. Irc3 modulates mtDNA metabolic intermediates by preferential binding and unwinding Holliday junctions and replication fork structures. Furthermore, we demonstrate that the loss of Irc3 can be complemented with mitochondrially targeted RecG of Escherichia coli. We suggest that Irc3 could support the stability of mtDNA by stimulating fork regression and branch migration or by inhibiting the formation of irregular branched molecules.
Figures
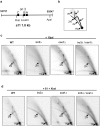
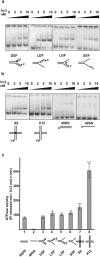
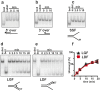
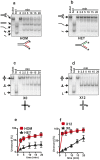
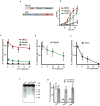
Similar articles
-
IRC3 Regulates Mitochondrial Translation in Response to Metabolic Cues in Saccharomyces cerevisiae.Mol Cell Biol. 2021 Oct 26;41(11):e0023321. doi: 10.1128/MCB.00233-21. Epub 2021 Aug 16. Mol Cell Biol. 2021. PMID: 34398681 Free PMC article.
-
Mitochondrial helicase Irc3 translocates along double-stranded DNA.FEBS Lett. 2017 Dec;591(23):3831-3841. doi: 10.1002/1873-3468.12903. Epub 2017 Nov 22. FEBS Lett. 2017. PMID: 29113022
-
Irc3 is a monomeric DNA branch point-binding helicase in mitochondria of the yeast Saccharomyces cerevisiae.FEBS Lett. 2020 Oct;594(19):3142-3155. doi: 10.1002/1873-3468.13893. Epub 2020 Aug 9. FEBS Lett. 2020. PMID: 32735705
-
Yeast and human mitochondrial helicases.Biochim Biophys Acta. 2013 Aug;1829(8):842-53. doi: 10.1016/j.bbagrm.2013.02.009. Epub 2013 Feb 27. Biochim Biophys Acta. 2013. PMID: 23454114 Review.
-
Maintenance and integrity of the mitochondrial genome: a plethora of nuclear genes in the budding yeast.Microbiol Mol Biol Rev. 2000 Jun;64(2):281-315. doi: 10.1128/MMBR.64.2.281-315.2000. Microbiol Mol Biol Rev. 2000. PMID: 10839818 Free PMC article. Review.
Cited by
-
Deletion of IRC19 Causes Defects in DNA Double-Strand Break Repair Pathways in Saccharomyces cerevisiae.J Microbiol. 2024 Sep;62(9):749-758. doi: 10.1007/s12275-024-00152-x. Epub 2024 Jul 12. J Microbiol. 2024. PMID: 38995433
-
IRC3 Regulates Mitochondrial Translation in Response to Metabolic Cues in Saccharomyces cerevisiae.Mol Cell Biol. 2021 Oct 26;41(11):e0023321. doi: 10.1128/MCB.00233-21. Epub 2021 Aug 16. Mol Cell Biol. 2021. PMID: 34398681 Free PMC article.
-
RecG controls DNA amplification at double-strand breaks and arrested replication forks.FEBS Lett. 2017 Apr;591(8):1101-1113. doi: 10.1002/1873-3468.12583. Epub 2017 Feb 28. FEBS Lett. 2017. PMID: 28155219 Free PMC article. Review.
References
Publication types
MeSH terms
Substances
LinkOut - more resources
Full Text Sources
Other Literature Sources
Molecular Biology Databases