Chemo-mechanical pushing of proteins along single-stranded DNA
- PMID: 27185951
- PMCID: PMC4896671
- DOI: 10.1073/pnas.1602878113
Chemo-mechanical pushing of proteins along single-stranded DNA
Abstract
Single-stranded (ss)DNA binding (SSB) proteins bind with high affinity to ssDNA generated during DNA replication, recombination, and repair; however, these SSBs must eventually be displaced from or reorganized along the ssDNA. One potential mechanism for reorganization is for an ssDNA translocase (ATP-dependent motor) to push the SSB along ssDNA. Here we use single molecule total internal reflection fluorescence microscopy to detect such pushing events. When Cy5-labeled Escherichia coli (Ec) SSB is bound to surface-immobilized 3'-Cy3-labeled ssDNA, a fluctuating FRET signal is observed, consistent with random diffusion of SSB along the ssDNA. Addition of Saccharomyces cerevisiae Pif1, a 5' to 3' ssDNA translocase, results in the appearance of isolated, irregularly spaced saw-tooth FRET spikes only in the presence of ATP. These FRET spikes result from translocase-induced directional (5' to 3') pushing of the SSB toward the 3' ssDNA end, followed by displacement of the SSB from the DNA end. Similar ATP-dependent pushing events, but in the opposite (3' to 5') direction, are observed with EcRep and EcUvrD (both 3' to 5' ssDNA translocases). Simulations indicate that these events reflect active pushing by the translocase. The ability of translocases to chemo-mechanically push heterologous SSB proteins along ssDNA provides a potential mechanism for reorganization and clearance of tightly bound SSBs from ssDNA.
Keywords: DNA motors; SF1 translocases; SSB proteins; dynamics.
Conflict of interest statement
The authors declare no conflict of interest.
Figures
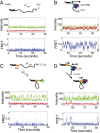
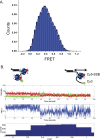
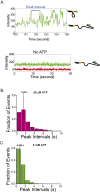
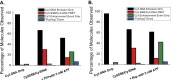
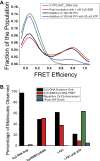
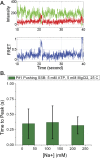
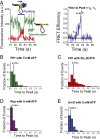
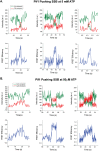
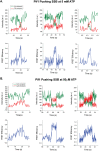
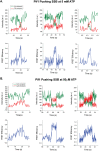
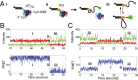
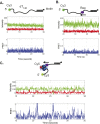
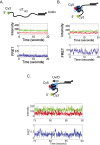
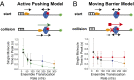
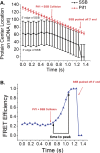
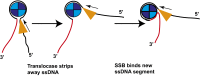
Similar articles
-
Single-molecule analysis of SSB dynamics on single-stranded DNA.Methods Mol Biol. 2012;922:85-100. doi: 10.1007/978-1-62703-032-8_5. Methods Mol Biol. 2012. PMID: 22976178
-
Molecular mechanism of DNA association with single-stranded DNA binding protein.Nucleic Acids Res. 2017 Dec 1;45(21):12125-12139. doi: 10.1093/nar/gkx917. Nucleic Acids Res. 2017. PMID: 29059392 Free PMC article.
-
"Helicase" Activity promoted through dynamic interactions between a ssDNA translocase and a diffusing SSB protein.Proc Natl Acad Sci U S A. 2023 Apr 11;120(15):e2216777120. doi: 10.1073/pnas.2216777120. Epub 2023 Apr 3. Proc Natl Acad Sci U S A. 2023. PMID: 37011199 Free PMC article.
-
Molecular insights into the prototypical single-stranded DNA-binding protein from E. coli.Crit Rev Biochem Mol Biol. 2024 Feb-Apr;59(1-2):99-127. doi: 10.1080/10409238.2024.2330372. Epub 2024 May 21. Crit Rev Biochem Mol Biol. 2024. PMID: 38770626 Review.
-
Escherichia coli single-stranded DNA-binding protein: multiple DNA-binding modes and cooperativities.Annu Rev Biochem. 1994;63:527-70. doi: 10.1146/annurev.bi.63.070194.002523. Annu Rev Biochem. 1994. PMID: 7979247 Review.
Cited by
-
Dynamics of E. coli single stranded DNA binding (SSB) protein-DNA complexes.Semin Cell Dev Biol. 2019 Feb;86:102-111. doi: 10.1016/j.semcdb.2018.03.017. Epub 2018 Mar 30. Semin Cell Dev Biol. 2019. PMID: 29588158 Free PMC article. Review.
-
Sequential eviction of crowded nucleoprotein complexes by the exonuclease RecBCD molecular motor.Proc Natl Acad Sci U S A. 2017 Aug 1;114(31):E6322-E6331. doi: 10.1073/pnas.1701368114. Epub 2017 Jul 17. Proc Natl Acad Sci U S A. 2017. PMID: 28716908 Free PMC article.
-
Regulation of T7 gp2.5 binding dynamics by its C-terminal tail, template conformation and sequence.Nucleic Acids Res. 2023 Jul 21;51(13):6540-6553. doi: 10.1093/nar/gkad485. Nucleic Acids Res. 2023. PMID: 37254785 Free PMC article.
-
A new twist on PIFE: photoisomerisation-related fluorescence enhancement.ArXiv [Preprint]. 2023 Jul 10:arXiv:2302.12455v2. ArXiv. 2023. Update in: Methods Appl Fluoresc. 2023 Oct 12;12(1). doi: 10.1088/2050-6120/acfb58. PMID: 36866225 Free PMC article. Updated. Preprint.
-
Replicative DNA polymerases promote active displacement of SSB proteins during lagging strand synthesis.Nucleic Acids Res. 2019 Jun 20;47(11):5723-5734. doi: 10.1093/nar/gkz249. Nucleic Acids Res. 2019. PMID: 30968132 Free PMC article.
References
Publication types
MeSH terms
Substances
Grants and funding
LinkOut - more resources
Full Text Sources
Other Literature Sources
Molecular Biology Databases