The cellular membrane as a mediator for small molecule interaction with membrane proteins
- PMID: 27163493
- PMCID: PMC4983535
- DOI: 10.1016/j.bbamem.2016.04.016
The cellular membrane as a mediator for small molecule interaction with membrane proteins
Abstract
The cellular membrane constitutes the first element that encounters a wide variety of molecular species to which a cell might be exposed. Hosting a large number of structurally and functionally diverse proteins associated with this key metabolic compartment, the membrane not only directly controls the traffic of various molecules in and out of the cell, it also participates in such diverse and important processes as signal transduction and chemical processing of incoming molecular species. In this article, we present a number of cases where details of interaction of small molecular species such as drugs with the membrane, which are often experimentally inaccessible, have been studied using advanced molecular simulation techniques. We have selected systems in which partitioning of the small molecule with the membrane constitutes a key step for its final biological function, often binding to and interacting with a protein associated with the membrane. These examples demonstrate that membrane partitioning is not only important for the overall distribution of drugs and other small molecules into different compartments of the body, it may also play a key role in determining the efficiency and the mode of interaction of the drug with its target protein. This article is part of a Special Issue entitled: Biosimulations edited by Ilpo Vattulainen and Tomasz Róg.
Keywords: Membrane; Membrane proteins; Molecular dynamics; Small molecules.
Copyright © 2016 Elsevier B.V. All rights reserved.
Figures
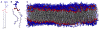
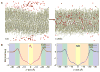
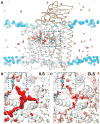
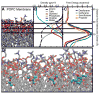
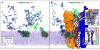
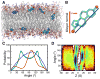
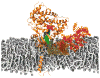
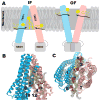
Similar articles
-
Closing the gap: The approach of optical and computational microscopy to uncover biomembrane organization.Biochim Biophys Acta. 2016 Oct;1858(10):2558-2568. doi: 10.1016/j.bbamem.2016.03.025. Epub 2016 Mar 31. Biochim Biophys Acta. 2016. PMID: 27039279 Review.
-
Lipid interaction sites on channels, transporters and receptors: Recent insights from molecular dynamics simulations.Biochim Biophys Acta. 2016 Oct;1858(10):2390-2400. doi: 10.1016/j.bbamem.2016.02.037. Epub 2016 Mar 3. Biochim Biophys Acta. 2016. PMID: 26946244 Free PMC article. Review.
-
Role of charged lipids in membrane structures - Insight given by simulations.Biochim Biophys Acta. 2016 Oct;1858(10):2322-2333. doi: 10.1016/j.bbamem.2016.03.016. Epub 2016 Mar 18. Biochim Biophys Acta. 2016. PMID: 27003126 Review.
-
Effects of protein crowding on membrane systems.Biochim Biophys Acta. 2016 Oct;1858(10):2441-2450. doi: 10.1016/j.bbamem.2015.12.021. Epub 2015 Dec 24. Biochim Biophys Acta. 2016. PMID: 26724385 Review.
-
Cell membranes: A subjective perspective.Biochim Biophys Acta. 2016 Oct;1858(10):2569-2572. doi: 10.1016/j.bbamem.2016.01.023. Epub 2016 Jan 29. Biochim Biophys Acta. 2016. PMID: 26827711
Cited by
-
Mechanistic Understanding from Molecular Dynamics in Pharmaceutical Research 2: Lipid Membrane in Drug Design.Pharmaceuticals (Basel). 2021 Oct 19;14(10):1062. doi: 10.3390/ph14101062. Pharmaceuticals (Basel). 2021. PMID: 34681286 Free PMC article. Review.
-
Mechanistic Understanding From Molecular Dynamics Simulation in Pharmaceutical Research 1: Drug Delivery.Front Mol Biosci. 2020 Nov 25;7:604770. doi: 10.3389/fmolb.2020.604770. eCollection 2020. Front Mol Biosci. 2020. PMID: 33330633 Free PMC article. Review.
-
The ecological roles of microbial lipopeptides: Where are we going?Comput Struct Biotechnol J. 2021 Mar 2;19:1400-1413. doi: 10.1016/j.csbj.2021.02.017. eCollection 2021. Comput Struct Biotechnol J. 2021. PMID: 33777336 Free PMC article. Review.
-
The interaction of steroids with phospholipid bilayers and membranes.Biophys Rev. 2021 Dec 2;14(1):163-179. doi: 10.1007/s12551-021-00918-2. eCollection 2022 Feb. Biophys Rev. 2021. PMID: 35340606 Free PMC article. Review.
-
Differential dynamics and direct interaction of bound ligands with lipids in multidrug transporter ABCG2.Proc Natl Acad Sci U S A. 2023 Jan 3;120(1):e2213437120. doi: 10.1073/pnas.2213437120. Epub 2022 Dec 29. Proc Natl Acad Sci U S A. 2023. PMID: 36580587 Free PMC article.
References
Publication types
MeSH terms
Substances
Grants and funding
LinkOut - more resources
Full Text Sources
Other Literature Sources