Analysis of the interacting partners eIF4F and 3'-CITE required for Melon necrotic spot virus cap-independent translation
- PMID: 27145354
- PMCID: PMC6638222
- DOI: 10.1111/mpp.12422
Analysis of the interacting partners eIF4F and 3'-CITE required for Melon necrotic spot virus cap-independent translation
Abstract
We have shown previously that the translation of Melon necrotic spot virus (MNSV, family Tombusviridae, genus Carmovirus) RNAs is controlled by a 3'-cap-independent translation enhancer (CITE), which is genetically and functionally dependent on the eukaryotic translation initiation factor (eIF) 4E. Here, we describe structural and functional analyses of the MNSV-Mα5 3'-CITE and its translation initiation factor partner. We first mapped the minimal 3'-CITE (Ma5TE) to a 45-nucleotide sequence, which consists of a stem-loop structure with two internal loops, similar to other I-shaped 3'-CITEs. UV crosslinking, followed by gel retardation assays, indicated that Ma5TE interacts in vitro with the complex formed by eIF4E + eIF4G980-1159 (eIF4Fp20 ), but not with each subunit alone or with eIF4E + eIF4G1003-1092 , suggesting binding either through interaction with eIF4E following a conformational change induced by its binding to eIF4G980-1159 , or through a double interaction with eIF4E and eIF4G980-1159 . Critical residues for this interaction reside in an internal bulge of Ma5TE, so that their mutation abolished binding to eIF4E + eIF4G1003-1092 and cap-independent translation. We also developed an in vivo system to test the effect of mutations in eIF4E in Ma5TE-driven cap-independent translation, showing that conserved amino acids in a positively charged RNA-binding motif around amino acid position 228, implicated in eIF4E-eIF4G binding or belonging to the cap-recognition pocket, are essential for cap-independent translation controlled by Ma5TE, and thus for the multiplication of MNSV.
Keywords: CITE; Carmovirus; RNA structure; Tombusviridae; cucurbit; translation enhancer; translation initiation.
© 2016 BSPP AND JOHN WILEY & SONS LTD.
Figures
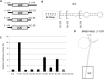
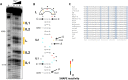
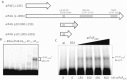
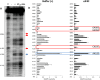
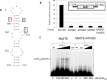
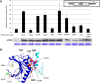
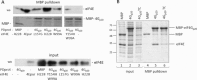
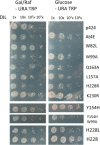
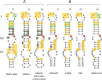
Similar articles
-
Structural and Functional Diversity of Plant Virus 3'-Cap-Independent Translation Enhancers (3'-CITEs).Front Plant Sci. 2017 Nov 29;8:2047. doi: 10.3389/fpls.2017.02047. eCollection 2017. Front Plant Sci. 2017. PMID: 29238357 Free PMC article. Review.
-
Mechanism of plant eIF4E-mediated resistance against a Carmovirus (Tombusviridae): cap-independent translation of a viral RNA controlled in cis by an (a)virulence determinant.Plant J. 2008 Dec;56(5):716-27. doi: 10.1111/j.1365-313X.2008.03630.x. Epub 2008 Jul 19. Plant J. 2008. PMID: 18643998
-
Structure of a viral cap-independent translation element that functions via high affinity binding to the eIF4E subunit of eIF4F.J Biol Chem. 2009 May 22;284(21):14189-202. doi: 10.1074/jbc.M808841200. Epub 2009 Mar 10. J Biol Chem. 2009. PMID: 19276085 Free PMC article.
-
Nicotiana benthamiana resistance to non-adapted Melon necrotic spot virus results from an incompatible interaction between virus RNA and translation initiation factor 4E.Plant J. 2011 May;66(3):492-501. doi: 10.1111/j.1365-313X.2011.04507.x. Epub 2011 Mar 7. Plant J. 2011. PMID: 21255163
-
Manipulation of the host translation initiation complex eIF4F by DNA viruses.Biochem Soc Trans. 2010 Dec;38(6):1511-6. doi: 10.1042/BST0381511. Biochem Soc Trans. 2010. PMID: 21118117 Review.
Cited by
-
Advances in Understanding the Mechanism of Cap-Independent Cucurbit Aphid-Borne Yellows Virus Protein Synthesis.Int J Mol Sci. 2023 Dec 18;24(24):17598. doi: 10.3390/ijms242417598. Int J Mol Sci. 2023. PMID: 38139425 Free PMC article.
-
Structural and Functional Diversity of Plant Virus 3'-Cap-Independent Translation Enhancers (3'-CITEs).Front Plant Sci. 2017 Nov 29;8:2047. doi: 10.3389/fpls.2017.02047. eCollection 2017. Front Plant Sci. 2017. PMID: 29238357 Free PMC article. Review.
-
Cap-Independent mRNA Translation in Germ Cells.Int J Mol Sci. 2019 Jan 5;20(1):173. doi: 10.3390/ijms20010173. Int J Mol Sci. 2019. PMID: 30621249 Free PMC article. Review.
-
Different RNA Elements Control Viral Protein Synthesis in Polerovirus Isolates Evolved in Separate Geographical Regions.Int J Mol Sci. 2022 Oct 19;23(20):12503. doi: 10.3390/ijms232012503. Int J Mol Sci. 2022. PMID: 36293360 Free PMC article.
-
Simultaneous CRISPR/Cas9-mediated editing of cassava eIF4E isoforms nCBP-1 and nCBP-2 reduces cassava brown streak disease symptom severity and incidence.Plant Biotechnol J. 2019 Feb;17(2):421-434. doi: 10.1111/pbi.12987. Epub 2018 Oct 5. Plant Biotechnol J. 2019. PMID: 30019807 Free PMC article.
References
-
- Aitken, C.E. and Lorsch, J.R. (2012) A mechanistic overview of translation initiation in eukaryotes. Nat. Struct. Mol. Biol. 19, 568–576. - PubMed
-
- Altmann, M. , Muller, P.P. , Pelletier, J. , Sonenberg, N. and Trachsel, H. (1989) A mammalian translation initiation factor can substitute for its yeast homologue in vivo. J. Biol. Chem. 264, 12 145–12 147. - PubMed
-
- Charron, C. , Nicolaï, M. , Gallois, J.L. , Robaglia, C. , Moury, B. , Palloix, A. and Caranta, C. (2008) Natural variation and functional analyses provide evidence for co‐evolution between plant eIF4E and potyviral VPg. Plant J. 54, 56–68. - PubMed
Publication types
MeSH terms
Substances
LinkOut - more resources
Full Text Sources
Other Literature Sources
Miscellaneous