RNA-Free and Ribonucleoprotein-Associated Influenza Virus Polymerases Directly Bind the Serine-5-Phosphorylated Carboxyl-Terminal Domain of Host RNA Polymerase II
- PMID: 27099314
- PMCID: PMC4907247
- DOI: 10.1128/JVI.00494-16
RNA-Free and Ribonucleoprotein-Associated Influenza Virus Polymerases Directly Bind the Serine-5-Phosphorylated Carboxyl-Terminal Domain of Host RNA Polymerase II
Abstract
Influenza viruses subvert the transcriptional machinery of their hosts to synthesize their own viral mRNA. Ongoing transcription by cellular RNA polymerase II (Pol II) is required for viral mRNA synthesis. By a process known as cap snatching, the virus steals short 5' capped RNA fragments from host capped RNAs and uses them to prime viral transcription. An interaction between the influenza A virus RNA polymerase and the C-terminal domain (CTD) of the large subunit of Pol II has been established, but the molecular details of this interaction remain unknown. We show here that the influenza virus ribonucleoprotein (vRNP) complex binds to the CTD of transcriptionally engaged Pol II. Furthermore, we provide evidence that the viral polymerase binds directly to the serine-5-phosphorylated form of the Pol II CTD, both in the presence and in the absence of viral RNA, and show that this interaction is conserved in evolutionarily distant influenza viruses. We propose a model in which direct binding of the viral RNA polymerase in the context of vRNPs to Pol II early in infection facilitates cap snatching, while we suggest that binding of free viral polymerase to Pol II late in infection may trigger Pol II degradation.
Importance: Influenza viruses cause yearly epidemics and occasional pandemics that pose a threat to human health, as well as represent a large economic burden to health care systems globally. Existing vaccines are not always effective, as they may not exactly match the circulating viruses. Furthermore, there are a limited number of antivirals available, and development of resistance to these is a concern. New measures to combat influenza are needed, but before they can be developed, it is necessary to better understand the molecular interactions between influenza viruses and their host cells. By providing further insights into the molecular details of how influenza viruses hijack the host transcriptional machinery, we aim to uncover novel targets for the development of antivirals.
Copyright © 2016 Martínez-Alonso et al.
Figures
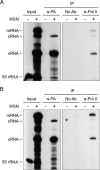
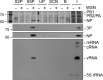
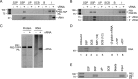
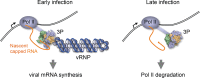
Similar articles
-
Structural and functional characterization of the interaction between the influenza A virus RNA polymerase and the CTD of host RNA polymerase II.J Virol. 2024 May 14;98(5):e0013824. doi: 10.1128/jvi.00138-24. Epub 2024 Apr 2. J Virol. 2024. PMID: 38563748 Free PMC article.
-
Interplay between Influenza Virus and the Host RNA Polymerase II Transcriptional Machinery.Trends Microbiol. 2019 May;27(5):398-407. doi: 10.1016/j.tim.2018.12.013. Epub 2019 Jan 11. Trends Microbiol. 2019. PMID: 30642766 Free PMC article. Review.
-
Type B and type A influenza polymerases have evolved distinct binding interfaces to recruit the RNA polymerase II CTD.PLoS Pathog. 2022 May 23;18(5):e1010328. doi: 10.1371/journal.ppat.1010328. eCollection 2022 May. PLoS Pathog. 2022. PMID: 35605026 Free PMC article.
-
The C-Terminal Domains of the PB2 Subunit of the Influenza A Virus RNA Polymerase Directly Interact with Cellular GTPase Rab11a.J Virol. 2022 Mar 9;96(5):e0197921. doi: 10.1128/jvi.01979-21. Epub 2022 Jan 12. J Virol. 2022. PMID: 35019720 Free PMC article.
-
Influenza virus RNA polymerase: insights into the mechanisms of viral RNA synthesis.Nat Rev Microbiol. 2016 Aug;14(8):479-93. doi: 10.1038/nrmicro.2016.87. Epub 2016 Jul 11. Nat Rev Microbiol. 2016. PMID: 27396566 Free PMC article. Review.
Cited by
-
The Influenza A Virus Replication Cycle: A Comprehensive Review.Viruses. 2024 Feb 19;16(2):316. doi: 10.3390/v16020316. Viruses. 2024. PMID: 38400091 Free PMC article. Review.
-
Structural and functional characterization of the interaction between the influenza A virus RNA polymerase and the CTD of host RNA polymerase II.J Virol. 2024 May 14;98(5):e0013824. doi: 10.1128/jvi.00138-24. Epub 2024 Apr 2. J Virol. 2024. PMID: 38563748 Free PMC article.
-
Efficient genome replication in influenza A virus requires NS2 and sequence beyond the canonical promoter.bioRxiv [Preprint]. 2024 Sep 10:2024.09.10.612348. doi: 10.1101/2024.09.10.612348. bioRxiv. 2024. PMID: 39314307 Free PMC article. Preprint.
-
A Mechanism for the Activation of the Influenza Virus Transcriptase.Mol Cell. 2018 Jun 21;70(6):1101-1110.e4. doi: 10.1016/j.molcel.2018.05.011. Epub 2018 Jun 14. Mol Cell. 2018. PMID: 29910112 Free PMC article.
-
Role of RNA Polymerase II Promoter-Proximal Pausing in Viral Transcription.Viruses. 2022 Sep 13;14(9):2029. doi: 10.3390/v14092029. Viruses. 2022. PMID: 36146833 Free PMC article. Review.
References
MeSH terms
Substances
Grants and funding
LinkOut - more resources
Full Text Sources
Other Literature Sources
Molecular Biology Databases
Miscellaneous