Intracellular tortuosity underlies slow cAMP diffusion in adult ventricular myocytes
- PMID: 27089919
- PMCID: PMC4872880
- DOI: 10.1093/cvr/cvw080
Intracellular tortuosity underlies slow cAMP diffusion in adult ventricular myocytes
Abstract
Aims: 3',5'-Cyclic adenosine monophosphate (cAMP) signals in the heart are often confined to concentration microdomains shaped by cAMP diffusion and enzymatic degradation. While the importance of phosphodiesterases (degradative enzymes) in sculpting cAMP microdomains is well established in cardiomyocytes, less is known about cAMP diffusivity (DcAMP) and factors affecting it. Many earlier studies have reported fast diffusivity, which argues against sharply defined microdomains.
Methods and results: [cAMP] dynamics in the cytoplasm of adult rat ventricular myocytes were imaged using a fourth generation genetically encoded FRET-based sensor. The [cAMP]-response to the addition and removal of isoproterenol (β-adrenoceptor agonist) quantified the rates of cAMP synthesis and degradation. To obtain a read out of DcAMP, a stable [cAMP] gradient was generated using a microfluidic device which delivered agonist to one half of the myocyte only. After accounting for phosphodiesterase activity, DcAMP was calculated to be 32 µm(2)/s; an order of magnitude lower than in water. Diffusivity was independent of the amount of cAMP produced. Saturating cAMP-binding sites with the analogue 6-Bnz-cAMP did not accelerate DcAMP, arguing against a role of buffering in restricting cAMP mobility. cAMP diffused at a comparable rate to chemically unrelated but similar sized molecules, arguing for a common physical cause of restricted diffusivity. Lower mitochondrial density and order in neonatal cardiac myocytes allowed for faster diffusion, demonstrating the importance of mitochondria as physical barriers to cAMP mobility.
Conclusion: In adult cardiac myocytes, tortuosity due to physical barriers, notably mitochondria, restricts cAMP diffusion to levels that are more compatible with microdomain signalling.
Keywords: Diffusion reaction; FRET sensor; Mathematical modelling; Microfluidics; cAMP microdomains.
© The Author 2016. Published by Oxford University Press on behalf of the European Society of Cardiology.
Figures
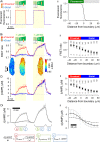
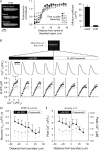
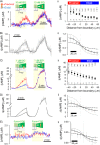
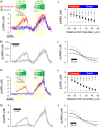
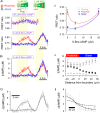
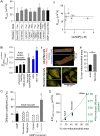
Similar articles
-
Heart failure leads to altered β2-adrenoceptor/cyclic adenosine monophosphate dynamics in the sarcolemmal phospholemman/Na,K ATPase microdomain.Cardiovasc Res. 2019 Mar 1;115(3):546-555. doi: 10.1093/cvr/cvy221. Cardiovasc Res. 2019. PMID: 30165515 Free PMC article.
-
Microdomain switch of cGMP-regulated phosphodiesterases leads to ANP-induced augmentation of β-adrenoceptor-stimulated contractility in early cardiac hypertrophy.Circ Res. 2015 Apr 10;116(8):1304-11. doi: 10.1161/CIRCRESAHA.116.306082. Epub 2015 Feb 16. Circ Res. 2015. PMID: 25688144
-
Cyclic AMP imaging in adult cardiac myocytes reveals far-reaching beta1-adrenergic but locally confined beta2-adrenergic receptor-mediated signaling.Circ Res. 2006 Nov 10;99(10):1084-91. doi: 10.1161/01.RES.0000250046.69918.d5. Epub 2006 Oct 12. Circ Res. 2006. PMID: 17038640
-
Compartmentalized cAMP signaling in cardiac ventricular myocytes.Cell Signal. 2022 Jan;89:110172. doi: 10.1016/j.cellsig.2021.110172. Epub 2021 Oct 20. Cell Signal. 2022. PMID: 34687901 Free PMC article. Review.
-
cAMP/PKA signaling compartmentalization in cardiomyocytes: Lessons from FRET-based biosensors.J Mol Cell Cardiol. 2019 Jun;131:112-121. doi: 10.1016/j.yjmcc.2019.04.020. Epub 2019 Apr 24. J Mol Cell Cardiol. 2019. PMID: 31028775 Review.
Cited by
-
PDE4 and mAKAPβ are nodal organizers of β2-ARs nuclear PKA signalling in cardiac myocytes.Cardiovasc Res. 2018 Sep 1;114(11):1499-1511. doi: 10.1093/cvr/cvy110. Cardiovasc Res. 2018. PMID: 29733383 Free PMC article.
-
cAMP Signaling Compartmentation: Adenylyl Cyclases as Anchors of Dynamic Signaling Complexes.Mol Pharmacol. 2018 Apr;93(4):270-276. doi: 10.1124/mol.117.110825. Epub 2017 Dec 7. Mol Pharmacol. 2018. PMID: 29217670 Free PMC article. Review.
-
Longitudinal diffusion barriers imposed by myofilaments and mitochondria in murine cardiac myocytes.J Gen Physiol. 2023 Oct 2;155(10):e202213329. doi: 10.1085/jgp.202213329. Epub 2023 Aug 9. J Gen Physiol. 2023. PMID: 37555782 Free PMC article.
-
Optical Mapping of cAMP Signaling at the Nanometer Scale.Cell. 2020 Sep 17;182(6):1519-1530.e17. doi: 10.1016/j.cell.2020.07.035. Epub 2020 Aug 25. Cell. 2020. PMID: 32846156 Free PMC article.
-
Na/K pump inactivation, subsarcolemmal Na measurements, and cytoplasmic ion turnover kinetics contradict restricted Na spaces in murine cardiac myocytes.J Gen Physiol. 2017 Jul 3;149(7):727-749. doi: 10.1085/jgp.201711780. Epub 2017 Jun 12. J Gen Physiol. 2017. PMID: 28606910 Free PMC article.
References
-
- Skalhegg BS, Tasken K. Specificity in the cAMP/PKA signaling pathway. Differential expression,regulation, and subcellular localization of subunits of PKA. Front Biosci 2000;5:D678–D693. - PubMed
Publication types
MeSH terms
Substances
Grants and funding
LinkOut - more resources
Full Text Sources
Other Literature Sources