Engineering of a novel cellulose-adherent cellulolytic Saccharomyces cerevisiae for cellulosic biofuel production
- PMID: 27079382
- PMCID: PMC4832201
- DOI: 10.1038/srep24550
Engineering of a novel cellulose-adherent cellulolytic Saccharomyces cerevisiae for cellulosic biofuel production
Abstract
Cellulosic biofuel is the subject of increasing attention. The main obstacle toward its economic feasibility is the recalcitrance of lignocellulose requiring large amount of enzyme to break. Several engineered yeast strains have been developed with cellulolytic activities to reduce the need for enzyme addition, but exhibiting limited effect. Here, we report the successful engineering of a cellulose-adherent Saccharomyces cerevisiae displaying four different synergistic cellulases on the cell surface. The cellulase-displaying yeast strain exhibited clear cell-to-cellulose adhesion and a "tearing" cellulose degradation pattern; the adhesion ability correlated with enhanced surface area and roughness of the target cellulose fibers, resulting in higher hydrolysis efficiency. The engineered yeast directly produced ethanol from rice straw despite a more than 40% decrease in the required enzyme dosage for high-density fermentation. Thus, improved cell-to-cellulose interactions provided a novel strategy for increasing cellulose hydrolysis, suggesting a mechanism for promoting the feasibility of cellulosic biofuel production.
Figures
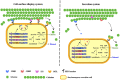
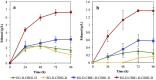
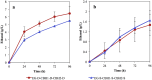
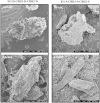
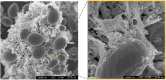
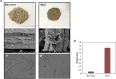
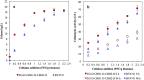
Similar articles
-
Improvement of ethanol production from crystalline cellulose via optimizing cellulase ratios in cellulolytic Saccharomyces cerevisiae.Biotechnol Bioeng. 2017 Jun;114(6):1201-1207. doi: 10.1002/bit.26252. Epub 2017 Mar 6. Biotechnol Bioeng. 2017. PMID: 28112385
-
Enhanced cellulase recovery without β-glucosidase supplementation for cellulosic ethanol production using an engineered strain and surfactant.Biotechnol Bioeng. 2017 Mar;114(3):543-551. doi: 10.1002/bit.26194. Epub 2016 Oct 10. Biotechnol Bioeng. 2017. PMID: 27696443
-
Improvement of cell-tethered cellulase activity in recombinant strains of Saccharomyces cerevisiae.Appl Microbiol Biotechnol. 2022 Sep;106(18):6347-6361. doi: 10.1007/s00253-022-12114-7. Epub 2022 Aug 11. Appl Microbiol Biotechnol. 2022. PMID: 35951080
-
Cellulolytic enzyme production and enzymatic hydrolysis for second-generation bioethanol production.Adv Biochem Eng Biotechnol. 2012;128:1-24. doi: 10.1007/10_2011_131. Adv Biochem Eng Biotechnol. 2012. PMID: 22231654 Review.
-
Engineering microbes for direct fermentation of cellulose to bioethanol.Crit Rev Biotechnol. 2018 Nov;38(7):1089-1105. doi: 10.1080/07388551.2018.1452891. Epub 2018 Apr 10. Crit Rev Biotechnol. 2018. PMID: 29631429 Review.
Cited by
-
Elucidating structure-performance relationships in whole-cell cooperative enzyme catalysis.Nat Catal. 2019;2(9):809-819. doi: 10.1038/s41929-019-0321-8. Epub 2019 Jul 22. Nat Catal. 2019. PMID: 33134840 Free PMC article.
-
Synergies in coupled hydrolysis and fermentation of cellulose using a Trichoderma reesei enzyme preparation and a recombinant Saccharomyces cerevisiae strain.World J Microbiol Biotechnol. 2017 Jul;33(7):140. doi: 10.1007/s11274-017-2308-4. Epub 2017 Jun 6. World J Microbiol Biotechnol. 2017. PMID: 28589508
-
A scalable peptide-GPCR language for engineering multicellular communication.Nat Commun. 2018 Nov 29;9(1):5057. doi: 10.1038/s41467-018-07610-2. Nat Commun. 2018. PMID: 30498215 Free PMC article.
-
The heterologous expression potential of an acid-tolerant Talaromyces pinophilus β-glucosidase in Saccharomyces cerevisiae.Folia Microbiol (Praha). 2018 Nov;63(6):725-734. doi: 10.1007/s12223-018-0613-4. Epub 2018 May 24. Folia Microbiol (Praha). 2018. PMID: 29797223
-
Cellulose-coated emulsion micro-particles self-assemble with yeasts for cellulose bio-conversion.Sci Rep. 2024 Mar 6;14(1):5499. doi: 10.1038/s41598-024-56204-0. Sci Rep. 2024. PMID: 38448579 Free PMC article.
References
-
- Sheehan J. Engineering direct conversion of CO2 to biofuel. Nat. Biotechnol. 27, 1128–1129 (2009). - PubMed
-
- Lynd L. R., Wyman C. E. & Gerngross T. U. Biocommodity Engineering. Biotechnol. Prog. 15, 777–793 (1999). - PubMed
-
- Himmel M. E. et al. Biomass recalcitrance: engineering plants and enzymes for biofuels production. Science 315, 804–807 (2007). - PubMed
-
- Schubert C. Can biofuels finally take center stage? Nat. Biotechnol. 24, 777–784 (2006). - PubMed
-
- van Zyl W. H., Lynd L. R., den Haan R. & McBride J. E. Consolidated bioprocessing for bioethanol production using Saccharomyces cerevisiae. Adv. Biochem. Eng. Biotechnol. 108, 205–235 (2007). - PubMed
Publication types
MeSH terms
Substances
LinkOut - more resources
Full Text Sources
Other Literature Sources