Controlled Drug Release and Chemotherapy Response in a Novel Acoustofluidic 3D Tumor Platform
- PMID: 27031786
- PMCID: PMC4889337
- DOI: 10.1002/smll.201503342
Controlled Drug Release and Chemotherapy Response in a Novel Acoustofluidic 3D Tumor Platform
Abstract
Overcoming transport barriers to delivery of therapeutic agents in tumors remains a major challenge. Focused ultrasound (FUS), in combination with modern nanomedicine drug formulations, offers the ability to maximize drug transport to tumor tissue while minimizing toxicity to normal tissue. This potential remains unfulfilled due to the limitations of current approaches in accurately assessing and quantifying how FUS modulates drug transport in solid tumors. A novel acoustofluidic platform is developed by integrating a physiologically relevant 3D microfluidic device and a FUS system with a closed-loop controller to study drug transport and assess the response of cancer cells to chemotherapy in real time using live cell microscopy. FUS-induced heating triggers local release of the chemotherapeutic agent doxorubicin from a liposomal carrier and results in higher cellular drug uptake in the FUS focal region. This differential drug uptake induces locally confined DNA damage and glioblastoma cell death in the 3D environment. The capabilities of acoustofluidics for accurate control of drug release and monitoring of localized cell response are demonstrated in a 3D in vitro tumor mode. This has important implications for developing novel strategies to deliver therapeutic agents directly to the tumor tissue while sparing healthy tissue.
Keywords: acoustofluidics; controlled release; drug delivery; focused ultrasound; tumor microenvironments.
© 2016 WILEY-VCH Verlag GmbH & Co. KGaA, Weinheim.
Figures
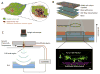
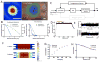
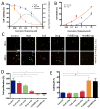
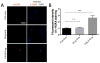
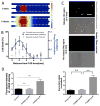
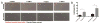
Similar articles
-
Coaxial nozzle-assisted 3D bioprinting with built-in microchannels for nutrients delivery.Biomaterials. 2015 Aug;61:203-15. doi: 10.1016/j.biomaterials.2015.05.031. Epub 2015 May 19. Biomaterials. 2015. PMID: 26004235
-
An optofluidic constriction chip for monitoring metastatic potential and drug response of cancer cells.Integr Biol (Camb). 2015 Apr;7(4):477-84. doi: 10.1039/c5ib00023h. Integr Biol (Camb). 2015. PMID: 25804890
-
Drug sensitivity of single cancer cells is predicted by changes in mass accumulation rate.Nat Biotechnol. 2016 Nov;34(11):1161-1167. doi: 10.1038/nbt.3697. Epub 2016 Oct 10. Nat Biotechnol. 2016. PMID: 27723727 Free PMC article.
-
Heralding a new paradigm in 3D tumor modeling.Biomaterials. 2016 Nov;108:197-213. doi: 10.1016/j.biomaterials.2016.08.052. Epub 2016 Sep 2. Biomaterials. 2016. PMID: 27639438 Free PMC article. Review.
-
Advanced targeted therapies in cancer: Drug nanocarriers, the future of chemotherapy.Eur J Pharm Biopharm. 2015 Jun;93:52-79. doi: 10.1016/j.ejpb.2015.03.018. Epub 2015 Mar 23. Eur J Pharm Biopharm. 2015. PMID: 25813885 Review.
Cited by
-
Current Challenges toward In Vitro Cellular Validation of Inorganic Nanoparticles.Bioconjug Chem. 2017 Jan 18;28(1):212-221. doi: 10.1021/acs.bioconjchem.6b00514. Epub 2016 Oct 19. Bioconjug Chem. 2017. PMID: 27709892 Free PMC article. Review.
-
Glioblastoma-on-a-chip construction and therapeutic applications.Front Oncol. 2023 Jul 12;13:1183059. doi: 10.3389/fonc.2023.1183059. eCollection 2023. Front Oncol. 2023. PMID: 37503321 Free PMC article. Review.
-
Caring for cells in microsystems: principles and practices of cell-safe device design and operation.Lab Chip. 2018 Nov 6;18(22):3333-3352. doi: 10.1039/c8lc00746b. Lab Chip. 2018. PMID: 30324208 Free PMC article. Review.
-
Targeted Cancer Therapy-on-A-Chip.Adv Healthc Mater. 2024 Nov;13(29):e2400833. doi: 10.1002/adhm.202400833. Epub 2024 Aug 5. Adv Healthc Mater. 2024. PMID: 39101627 Free PMC article. Review.
-
Cancer-on-a-chip systems at the frontier of nanomedicine.Drug Discov Today. 2017 Sep;22(9):1392-1399. doi: 10.1016/j.drudis.2017.03.011. Epub 2017 Apr 5. Drug Discov Today. 2017. PMID: 28390929 Free PMC article. Review.
References
-
- Olson JJ, Nayak L, Ormond DR, Wen PY, Kalkanis SN, Ryken TC, Committee ACJG. Journal of neuro-oncology. 2014;118(3):557–99. - PubMed
Publication types
MeSH terms
Substances
Grants and funding
LinkOut - more resources
Full Text Sources
Other Literature Sources