β-Arrestin biosensors reveal a rapid, receptor-dependent activation/deactivation cycle
- PMID: 27007855
- PMCID: PMC5157050
- DOI: 10.1038/nature17198
β-Arrestin biosensors reveal a rapid, receptor-dependent activation/deactivation cycle
Abstract
(β-)Arrestins are important regulators of G-protein-coupled receptors (GPCRs). They bind to active, phosphorylated GPCRs and thereby shut off 'classical' signalling to G proteins, trigger internalization of GPCRs via interaction with the clathrin machinery and mediate signalling via 'non-classical' pathways. In addition to two visual arrestins that bind to rod and cone photoreceptors (termed arrestin1 and arrestin4), there are only two (non-visual) β-arrestin proteins (β-arrestin1 and β-arrestin2, also termed arrestin2 and arrestin3), which regulate hundreds of different (non-visual) GPCRs. Binding of these proteins to GPCRs usually requires the active form of the receptors plus their phosphorylation by G-protein-coupled receptor kinases (GRKs). The binding of receptors or their carboxy terminus as well as certain truncations induce active conformations of (β-)arrestins that have recently been solved by X-ray crystallography. Here we investigate both the interaction of β-arrestin with GPCRs, and the β-arrestin conformational changes in real time and in living human cells, using a series of fluorescence resonance energy transfer (FRET)-based β-arrestin2 biosensors. We observe receptor-specific patterns of conformational changes in β-arrestin2 that occur rapidly after the receptor-β-arrestin2 interaction. After agonist removal, these changes persist for longer than the direct receptor interaction. Our data indicate a rapid, receptor-type-specific, two-step binding and activation process between GPCRs and β-arrestins. They further indicate that β-arrestins remain active after dissociation from receptors, allowing them to remain at the cell surface and presumably signal independently. Thus, GPCRs trigger a rapid, receptor-specific activation/deactivation cycle of β-arrestins, which permits their active signalling.
Figures
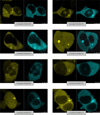
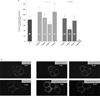
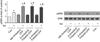
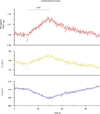
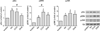
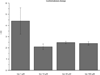
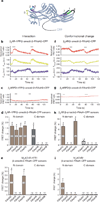
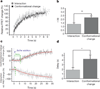
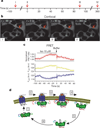
Similar articles
-
Beta-arrestin1 and beta-arrestin2 are differentially required for phosphorylation-dependent and -independent internalization of delta-opioid receptors.J Neurochem. 2005 Oct;95(1):169-78. doi: 10.1111/j.1471-4159.2005.03352.x. J Neurochem. 2005. PMID: 16181421
-
The conformational signature of β-arrestin2 predicts its trafficking and signalling functions.Nature. 2016 Mar 31;531(7596):665-8. doi: 10.1038/nature17154. Epub 2016 Mar 23. Nature. 2016. PMID: 27007854 Free PMC article.
-
Structure of active β-arrestin-1 bound to a G-protein-coupled receptor phosphopeptide.Nature. 2013 May 2;497(7447):137-41. doi: 10.1038/nature12120. Epub 2013 Apr 21. Nature. 2013. PMID: 23604254 Free PMC article.
-
β-Arrestins and G protein-coupled receptor trafficking.Methods Enzymol. 2013;521:91-108. doi: 10.1016/B978-0-12-391862-8.00005-3. Methods Enzymol. 2013. PMID: 23351735 Review.
-
β-arrestins and G protein-coupled receptor trafficking.Handb Exp Pharmacol. 2014;219:173-86. doi: 10.1007/978-3-642-41199-1_9. Handb Exp Pharmacol. 2014. PMID: 24292830 Free PMC article. Review.
Cited by
-
β-arrestin1 and 2 exhibit distinct phosphorylation-dependent conformations when coupling to the same GPCR in living cells.Nat Commun. 2022 Sep 26;13(1):5638. doi: 10.1038/s41467-022-33307-8. Nat Commun. 2022. PMID: 36163356 Free PMC article.
-
Key phosphorylation sites in GPCRs orchestrate the contribution of β-Arrestin 1 in ERK1/2 activation.EMBO Rep. 2020 Sep 3;21(9):e49886. doi: 10.15252/embr.201949886. Epub 2020 Jul 26. EMBO Rep. 2020. PMID: 32715625 Free PMC article.
-
Single hormone or synthetic agonist induces Gs/Gi coupling selectivity of EP receptors via distinct binding modes and propagating paths.Proc Natl Acad Sci U S A. 2023 Jul 25;120(30):e2216329120. doi: 10.1073/pnas.2216329120. Epub 2023 Jul 21. Proc Natl Acad Sci U S A. 2023. PMID: 37478163 Free PMC article.
-
Plasma membrane preassociation drives β-arrestin coupling to receptors and activation.Cell. 2023 May 11;186(10):2238-2255.e20. doi: 10.1016/j.cell.2023.04.018. Epub 2023 May 4. Cell. 2023. PMID: 37146613 Free PMC article.
-
Using nanoBRET and CRISPR/Cas9 to monitor proximity to a genome-edited protein in real-time.Sci Rep. 2017 Jun 9;7(1):3187. doi: 10.1038/s41598-017-03486-2. Sci Rep. 2017. PMID: 28600500 Free PMC article.
References
-
- Lohse MJ, Hoffmann C. Arrestin interactions with G protein-coupled receptors. Handb. Exp. Pharmacol. 2014;219:15–56. - PubMed
-
- Lohse MJ, Benovic JL, Codina J, Caron MG, Lefkowitz RJ. β-Arrestin: a protein that regulates β-adrenergic receptor function. Science. 1990;248:1547–1550. - PubMed
-
- Goodman OB, et al. β-Arrestin acts as a clathrin adaptor in endocytosis of the β2-adrenergic receptor. Nature. 1996;383:447–450. - PubMed
Publication types
MeSH terms
Substances
Grants and funding
LinkOut - more resources
Full Text Sources
Other Literature Sources