Structure of promoter-bound TFIID and model of human pre-initiation complex assembly
- PMID: 27007846
- PMCID: PMC4856295
- DOI: 10.1038/nature17394
Structure of promoter-bound TFIID and model of human pre-initiation complex assembly
Erratum in
-
Corrigendum: Structure of promoter-bound TFIID and model of human pre-initiation complex assembly.Nature. 2016 Aug 4;536(7614):112. doi: 10.1038/nature17984. Epub 2016 Apr 20. Nature. 2016. PMID: 27096372 No abstract available.
Abstract
The general transcription factor IID (TFIID) plays a central role in the initiation of RNA polymerase II (Pol II)-dependent transcription by nucleating pre-initiation complex (PIC) assembly at the core promoter. TFIID comprises the TATA-binding protein (TBP) and 13 TBP-associated factors (TAF1-13), which specifically interact with a variety of core promoter DNA sequences. Here we present the structure of human TFIID in complex with TFIIA and core promoter DNA, determined by single-particle cryo-electron microscopy at sub-nanometre resolution. All core promoter elements are contacted by subunits of TFIID, with TAF1 and TAF2 mediating major interactions with the downstream promoter. TFIIA bridges the TBP-TATA complex with lobe B of TFIID. We also present the cryo-electron microscopy reconstruction of a fully assembled human TAF-less PIC. Superposition of common elements between the two structures provides novel insights into the general role of TFIID in promoter recognition, PIC assembly, and transcription initiation.
Conflict of interest statement
The authors declare no competing financial interests.
Figures
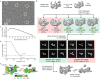
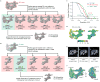
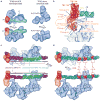
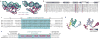
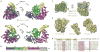
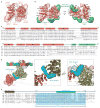
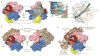
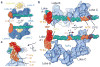
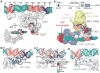
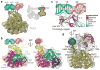
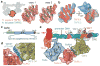
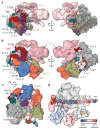
Similar articles
-
A transcription factor IIA-binding site differentially regulates RNA polymerase II-mediated transcription in a promoter context-dependent manner.J Biol Chem. 2017 Jul 14;292(28):11873-11885. doi: 10.1074/jbc.M116.770412. Epub 2017 May 24. J Biol Chem. 2017. PMID: 28539359 Free PMC article.
-
Cryo-EM reveals promoter DNA binding and conformational flexibility of the general transcription factor TFIID.Structure. 2009 Nov 11;17(11):1442-52. doi: 10.1016/j.str.2009.09.007. Structure. 2009. PMID: 19913479
-
Structural insights into assembly of transcription preinitiation complex.Curr Opin Struct Biol. 2022 Aug;75:102404. doi: 10.1016/j.sbi.2022.102404. Epub 2022 Jun 11. Curr Opin Struct Biol. 2022. PMID: 35700575 Review.
-
Structure of human TFIID and mechanism of TBP loading onto promoter DNA.Science. 2018 Dec 21;362(6421):eaau8872. doi: 10.1126/science.aau8872. Epub 2018 Nov 15. Science. 2018. PMID: 30442764 Free PMC article.
-
Promoter Recognition: Putting TFIID on the Spot.Trends Cell Biol. 2019 Sep;29(9):752-763. doi: 10.1016/j.tcb.2019.06.004. Epub 2019 Jul 9. Trends Cell Biol. 2019. PMID: 31300188 Review.
Cited by
-
Mechanisms and Functions of the RNA Polymerase II General Transcription Machinery during the Transcription Cycle.Biomolecules. 2024 Feb 1;14(2):176. doi: 10.3390/biom14020176. Biomolecules. 2024. PMID: 38397413 Free PMC article. Review.
-
Organization and regulation of gene transcription.Nature. 2019 Sep;573(7772):45-54. doi: 10.1038/s41586-019-1517-4. Epub 2019 Aug 28. Nature. 2019. PMID: 31462772 Review.
-
Epigenetics and transcription regulation during eukaryotic diversification: the saga of TFIID.Genes Dev. 2019 Aug 1;33(15-16):888-902. doi: 10.1101/gad.300475.117. Epub 2019 May 23. Genes Dev. 2019. PMID: 31123066 Free PMC article.
-
Structure and mechanism of the RNA polymerase II transcription machinery.Genes Dev. 2020 Apr 1;34(7-8):465-488. doi: 10.1101/gad.335679.119. Genes Dev. 2020. PMID: 32238450 Free PMC article. Review.
-
Eukaryotic core promoters and the functional basis of transcription initiation.Nat Rev Mol Cell Biol. 2018 Oct;19(10):621-637. doi: 10.1038/s41580-018-0028-8. Nat Rev Mol Cell Biol. 2018. PMID: 29946135 Free PMC article. Review.
References
-
- Thomas MC, Chiang CM. The general transcription machinery and general cofactors. Critical reviews in biochemistry and molecular biology. 2006;41:105–178. - PubMed
-
- Buratowski S, Hahn S, Guarente L, Sharp PA. Five intermediate complexes in transcription initiation by RNA polymerase II. Cell. 1989;56:549–561. - PubMed
-
- Burley SK, Roeder RG. Biochemistry and structural biology of transcription factor IID (TFIID) Annual review of biochemistry. 1996;65:769–799. - PubMed
-
- Albright SR, Tjian R. TAFs revisited: more data reveal new twists and confirm old ideas. Gene. 2000;242:1–13. - PubMed
Publication types
MeSH terms
Substances
Associated data
- Actions
Grants and funding
LinkOut - more resources
Full Text Sources
Other Literature Sources
Molecular Biology Databases