Mechanosensory and ATP Release Deficits following Keratin14-Cre-Mediated TRPA1 Deletion Despite Absence of TRPA1 in Murine Keratinocytes
- PMID: 26978657
- PMCID: PMC4792390
- DOI: 10.1371/journal.pone.0151602
Mechanosensory and ATP Release Deficits following Keratin14-Cre-Mediated TRPA1 Deletion Despite Absence of TRPA1 in Murine Keratinocytes
Abstract
Keratinocytes are the first cells that come into direct contact with external tactile stimuli; however, their role in touch transduction in vivo is not clear. The ion channel Transient Receptor Potential Ankyrin 1 (TRPA1) is essential for some mechanically-gated currents in sensory neurons, amplifies mechanical responses after inflammation, and has been reported to be expressed in human and mouse skin. Other reports have not detected Trpa1 mRNA transcripts in human or mouse epidermis. Therefore, we set out to determine whether selective deletion of Trpa1 from keratinocytes would impact mechanosensation. We generated K14Cre-Trpa1fl/fl mice lacking TRPA1 in K14-expressing cells, including keratinocytes. Surprisingly, Trpa1 transcripts were very poorly detected in epidermis of these mice or in controls, and detection was minimal enough to preclude observation of Trpa1 mRNA knockdown in the K14Cre-Trpa1fl/fl mice. Unexpectedly, these K14Cre-Trpa1fl/fl mice nonetheless exhibited a pronounced deficit in mechanosensitivity at the behavioral and primary afferent levels, and decreased mechanically-evoked ATP release from skin. Overall, while these data suggest that the intended targeted deletion of Trpa1 from keratin 14-expressing cells of the epidermis induces functional deficits in mechanotransduction and ATP release, these deficits are in fact likely due to factors other than reduction of Trpa1 expression in adult mouse keratinocytes because they express very little, if any, Trpa1.
Conflict of interest statement
Figures
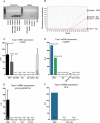
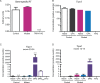
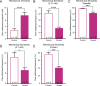
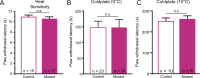
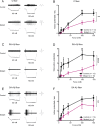
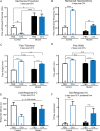
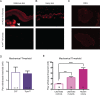
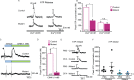
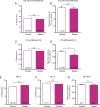
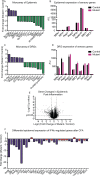
Similar articles
-
Sensory Neuron-Specific Deletion of TRPA1 Results in Mechanical Cutaneous Sensory Deficits.eNeuro. 2017 Mar 13;4(1):ENEURO.0069-16.2017. doi: 10.1523/ENEURO.0069-16.2017. eCollection 2017 Jan-Feb. eNeuro. 2017. PMID: 28303259 Free PMC article.
-
TRPA1 modulates mechanotransduction in cutaneous sensory neurons.J Neurosci. 2009 Apr 15;29(15):4808-19. doi: 10.1523/JNEUROSCI.5380-08.2009. J Neurosci. 2009. PMID: 19369549 Free PMC article.
-
Environmental cold exposure increases blood flow and affects pain sensitivity in the knee joints of CFA-induced arthritic mice in a TRPA1-dependent manner.Arthritis Res Ther. 2016 Jan 11;18:7. doi: 10.1186/s13075-015-0905-x. Arthritis Res Ther. 2016. PMID: 26754745 Free PMC article.
-
Mammalian Transient Receptor Potential TRPA1 Channels: From Structure to Disease.Physiol Rev. 2020 Apr 1;100(2):725-803. doi: 10.1152/physrev.00005.2019. Epub 2019 Oct 31. Physiol Rev. 2020. PMID: 31670612 Review.
-
Mini-review: The nociceptive sensory functions of the polymodal receptor Transient Receptor Potential Ankyrin Type 1 (TRPA1).Neurosci Lett. 2021 Nov 1;764:136286. doi: 10.1016/j.neulet.2021.136286. Epub 2021 Oct 5. Neurosci Lett. 2021. PMID: 34624396 Review.
Cited by
-
The Role of TRPA1 in Skin Physiology and Pathology.Int J Mol Sci. 2021 Mar 17;22(6):3065. doi: 10.3390/ijms22063065. Int J Mol Sci. 2021. PMID: 33802836 Free PMC article. Review.
-
A Novel Model of Cancer-Induced Peripheral Neuropathy and the Role of TRPA1 in Pain Transduction.Pain Res Manag. 2017 Dec 28;2017:3517207. doi: 10.1155/2017/3517207. eCollection 2017. Pain Res Manag. 2017. PMID: 30510606 Free PMC article.
-
Keratinocytes contribute to normal cold and heat sensation.Elife. 2020 Jul 30;9:e58625. doi: 10.7554/eLife.58625. Elife. 2020. PMID: 32729832 Free PMC article.
-
STIM1 thermosensitivity defines the optimal preference temperature for warm sensation in mice.Cell Res. 2019 Feb;29(2):95-109. doi: 10.1038/s41422-018-0129-0. Epub 2019 Jan 3. Cell Res. 2019. PMID: 30607017 Free PMC article.
-
Multimodal roles of transient receptor potential channel activation in inducing pathological tissue scarification.Front Immunol. 2023 Aug 29;14:1237992. doi: 10.3389/fimmu.2023.1237992. eCollection 2023. Front Immunol. 2023. PMID: 37705977 Free PMC article. Review.
References
-
- Zylka MJ, Rice FL, Anderson DJ. Topographically distinct epidermal nociceptive circuits revealed by axonal tracers targeted to Mrgprd. Neuron. 2005;45(1):17–25. - PubMed
Publication types
MeSH terms
Substances
Grants and funding
LinkOut - more resources
Full Text Sources
Other Literature Sources
Molecular Biology Databases