Communication in Neural Circuits: Tools, Opportunities, and Challenges
- PMID: 26967281
- PMCID: PMC5725393
- DOI: 10.1016/j.cell.2016.02.027
Communication in Neural Circuits: Tools, Opportunities, and Challenges
Abstract
Communication, the effective delivery of information, is fundamental to life across all scales and species. Nervous systems (by necessity) may be most specifically adapted among biological tissues for high rate and complexity of information transmitted, and thus, the properties of neural tissue and principles of its organization into circuits may illuminate capabilities and limitations of biological communication. Here, we consider recent developments in tools for studying neural circuits with particular attention to defining neuronal cell types by input and output information streams--i.e., by how they communicate. Complementing approaches that define cell types by virtue of genetic promoter/enhancer properties, this communication-based approach to defining cell types operationally by input/output (I/O) relationships links structure and function, resolves difficulties associated with single-genetic-feature definitions, leverages technology for observing and testing significance of precisely these I/O relationships in intact brains, and maps onto processes through which behavior may be adapted during development, experience, and evolution.
Copyright © 2016 Elsevier Inc. All rights reserved.
Figures
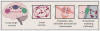
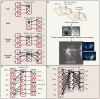
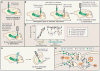
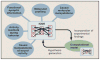
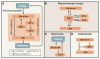
Similar articles
-
Optogenetic dissection of neural circuits underlying emotional valence and motivated behaviors.Brain Res. 2013 May 20;1511:73-92. doi: 10.1016/j.brainres.2012.11.001. Epub 2012 Nov 8. Brain Res. 2013. PMID: 23142759 Free PMC article. Review.
-
Light Up the Brain: The Application of Optogenetics in Cell-Type Specific Dissection of Mouse Brain Circuits.Front Neural Circuits. 2020 Apr 24;14:18. doi: 10.3389/fncir.2020.00018. eCollection 2020. Front Neural Circuits. 2020. PMID: 32390806 Free PMC article. Review.
-
Engineering Aspects of Olfaction.In: Persaud KC, Marco S, Gutiérrez-Gálvez A, editors. Neuromorphic Olfaction. Boca Raton (FL): CRC Press/Taylor & Francis; 2013. Chapter 1. In: Persaud KC, Marco S, Gutiérrez-Gálvez A, editors. Neuromorphic Olfaction. Boca Raton (FL): CRC Press/Taylor & Francis; 2013. Chapter 1. PMID: 26042329 Free Books & Documents. Review.
-
Intact-Brain Analyses Reveal Distinct Information Carried by SNc Dopamine Subcircuits.Cell. 2015 Jul 30;162(3):635-47. doi: 10.1016/j.cell.2015.07.014. Cell. 2015. PMID: 26232229 Free PMC article.
-
Genetic approaches to neural circuits in the mouse.Annu Rev Neurosci. 2013 Jul 8;36:183-215. doi: 10.1146/annurev-neuro-062012-170307. Epub 2013 May 17. Annu Rev Neurosci. 2013. PMID: 23682658 Review.
Cited by
-
Animal Models for OCD Research.Curr Top Behav Neurosci. 2021;49:55-96. doi: 10.1007/7854_2020_196. Curr Top Behav Neurosci. 2021. PMID: 33763819 Review.
-
A Student's Guide to Neural Circuit Tracing.Front Neurosci. 2019 Aug 27;13:897. doi: 10.3389/fnins.2019.00897. eCollection 2019. Front Neurosci. 2019. PMID: 31507369 Free PMC article. Review.
-
Q&A: How can advances in tissue clearing and optogenetics contribute to our understanding of normal and diseased biology?BMC Biol. 2017 Sep 25;15(1):87. doi: 10.1186/s12915-017-0421-3. BMC Biol. 2017. PMID: 28946882 Free PMC article.
-
Single-Cell RNA Sequencing: Unraveling the Brain One Cell at a Time.Trends Mol Med. 2017 Jun;23(6):563-576. doi: 10.1016/j.molmed.2017.04.006. Epub 2017 May 10. Trends Mol Med. 2017. PMID: 28501348 Free PMC article. Review.
-
Editorial: Unveiling the structure and function of brain microcircuits: Experiments, algorithms and simulations.Front Neural Circuits. 2022 Aug 12;16:991137. doi: 10.3389/fncir.2022.991137. eCollection 2022. Front Neural Circuits. 2022. PMID: 36034335 Free PMC article. No abstract available.
References
-
- Alexander GE, DeLong MR, Strick PL. Parallel organization of functionally segregated circuits linking basal ganglia and cortex. Annu Rev Neurosci. 1986;9:357–381. - PubMed
-
- Ballesteros-Yáñez I, Benavides-Piccione R, Elston GN, Yuste R, DeFelipe J. Density and morphology of dendritic spines in mouse neocortex. Neuroscience. 2006;138:403–409. - PubMed
Publication types
MeSH terms
Grants and funding
LinkOut - more resources
Full Text Sources
Other Literature Sources