Nociceptin/Orphanin FQ Receptor Structure, Signaling, Ligands, Functions, and Interactions with Opioid Systems
- PMID: 26956246
- PMCID: PMC4813427
- DOI: 10.1124/pr.114.009209
Nociceptin/Orphanin FQ Receptor Structure, Signaling, Ligands, Functions, and Interactions with Opioid Systems
Abstract
The NOP receptor (nociceptin/orphanin FQ opioid peptide receptor) is the most recently discovered member of the opioid receptor family and, together with its endogenous ligand, N/OFQ, make up the fourth members of the opioid receptor and opioid peptide family. Because of its more recent discovery, an understanding of the cellular and behavioral actions induced by NOP receptor activation are less well developed than for the other members of the opioid receptor family. All of these factors are important because NOP receptor activation has a clear modulatory role on mu opioid receptor-mediated actions and thereby affects opioid analgesia, tolerance development, and reward. In addition to opioid modulatory actions, NOP receptor activation has important effects on motor function and other physiologic processes. This review discusses how NOP pharmacology intersects, contrasts, and interacts with the mu opioid receptor in terms of tertiary structure and mechanism of receptor activation; location of receptors in the central nervous system; mechanisms of desensitization and downregulation; cellular actions; intracellular signal transduction pathways; and behavioral actions with respect to analgesia, tolerance, dependence, and reward. This is followed by a discussion of the agonists and antagonists that have most contributed to our current knowledge. Because NOP receptors are highly expressed in brain and spinal cord and NOP receptor activation sometimes synergizes with mu receptor-mediated actions and sometimes opposes them, an understanding of NOP receptor pharmacology in the context of these interactions with the opioid receptors will be crucial to the development of novel therapeutics that engage the NOP receptor.
U.S. Government work not protected by U.S. copyright.
Figures
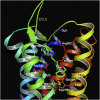
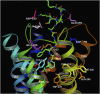
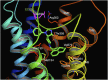
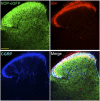
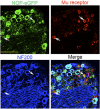
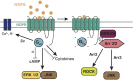
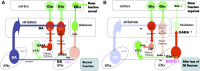
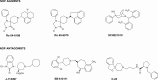
Similar articles
-
Cellular mechanisms of nociceptin/orphanin FQ (N/OFQ) peptide (NOP) receptor regulation and heterologous regulation by N/OFQ.Mol Pharmacol. 2013 May;83(5):907-18. doi: 10.1124/mol.112.084632. Epub 2013 Feb 8. Mol Pharmacol. 2013. PMID: 23395957 Free PMC article. Review.
-
The biology of Nociceptin/Orphanin FQ (N/OFQ) related to obesity, stress, anxiety, mood, and drug dependence.Pharmacol Ther. 2014 Mar;141(3):283-99. doi: 10.1016/j.pharmthera.2013.10.011. Epub 2013 Nov 1. Pharmacol Ther. 2014. PMID: 24189487 Free PMC article. Review.
-
Structure of the nociceptin/orphanin FQ receptor in complex with a peptide mimetic.Nature. 2012 May 16;485(7398):395-9. doi: 10.1038/nature11085. Nature. 2012. PMID: 22596163 Free PMC article.
-
Potential of Nociceptin/Orphanin FQ Peptide Analogs for Drug Development.Chem Biodivers. 2021 Jan;18(1):e2000871. doi: 10.1002/cbdv.202000871. Epub 2020 Dec 22. Chem Biodivers. 2021. PMID: 33351271 Review.
-
Pharmacological characterization of nociceptin/orphanin FQ receptors, a novel opioid receptor family, in the midbrain periaqueductal gray.Ann N Y Acad Sci. 2004 Oct;1025:398-403. doi: 10.1196/annals.1316.049. Ann N Y Acad Sci. 2004. PMID: 15542742 Review.
Cited by
-
Extended amygdala-parabrachial circuits alter threat assessment and regulate feeding.Sci Adv. 2021 Feb 26;7(9):eabd3666. doi: 10.1126/sciadv.abd3666. Print 2021 Feb. Sci Adv. 2021. PMID: 33637526 Free PMC article.
-
(L)-Monomethyl Tyrosine (Mmt): New Synthetic Strategy via Bulky 'Forced-Traceless' Regioselective Pd-Catalyzed C(sp2)-H Activation.Pharmaceuticals (Basel). 2023 Nov 10;16(11):1592. doi: 10.3390/ph16111592. Pharmaceuticals (Basel). 2023. PMID: 38004457 Free PMC article.
-
Rostromedial tegmental nucleus nociceptin/orphanin FQ (N/OFQ) signaling regulates anxiety- and depression-like behaviors in alcohol withdrawn rats.Neuropsychopharmacology. 2023 May;48(6):908-919. doi: 10.1038/s41386-022-01482-3. Epub 2022 Nov 3. Neuropsychopharmacology. 2023. PMID: 36329156 Free PMC article.
-
Epigenetic Modulation of Opioid Receptors by Drugs of Abuse.Int J Mol Sci. 2022 Oct 5;23(19):11804. doi: 10.3390/ijms231911804. Int J Mol Sci. 2022. PMID: 36233105 Free PMC article. Review.
-
Pain, Motivation, Migraine, and the Microbiome: New Frontiers for Opioid Systems and Disease.Mol Pharmacol. 2020 Oct;98(4):433-444. doi: 10.1124/mol.120.119438. Epub 2020 Jul 27. Mol Pharmacol. 2020. PMID: 32958571 Free PMC article. Review.
References
-
- Aguila B, Simaan M, Laporte SA. (2011) Study of G protein-coupled receptor/β-arrestin interactions within endosomes using FRAP. Methods Mol Biol 756:371–380. - PubMed
-
- Akuzawa N, Takeda S, Ishiguro M. (2007) Structural modelling and mutation analysis of a nociceptin receptor and its ligand complexes. J Biochem 141:907–916. - PubMed
Publication types
MeSH terms
Substances
Grants and funding
LinkOut - more resources
Full Text Sources
Other Literature Sources
Research Materials