Dynamic Protein Interaction Networks and New Structural Paradigms in Signaling
- PMID: 26922996
- PMCID: PMC5342629
- DOI: 10.1021/acs.chemrev.5b00548
Dynamic Protein Interaction Networks and New Structural Paradigms in Signaling
Abstract
Understanding signaling and other complex biological processes requires elucidating the critical roles of intrinsically disordered proteins (IDPs) and regions (IDRs), which represent ∼30% of the proteome and enable unique regulatory mechanisms. In this review, we describe the structural heterogeneity of disordered proteins that underpins these mechanisms and the latest progress in obtaining structural descriptions of conformational ensembles of disordered proteins that are needed for linking structure and dynamics to function. We describe the diverse interactions of IDPs that can have unusual characteristics such as "ultrasensitivity" and "regulated folding and unfolding". We also summarize the mounting data showing that large-scale assembly and protein phase separation occurs within a variety of signaling complexes and cellular structures. In addition, we discuss efforts to therapeutically target disordered proteins with small molecules. Overall, we interpret the remodeling of disordered state ensembles due to binding and post-translational modifications within an expanded framework for allostery that provides significant insights into how disordered proteins transmit biological information.
Figures
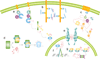
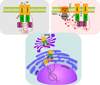
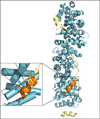
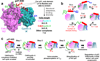
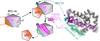
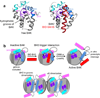
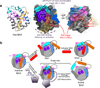
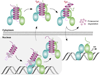
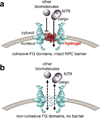
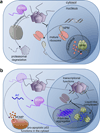
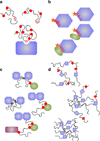
Similar articles
-
Dancing Protein Clouds: The Strange Biology and Chaotic Physics of Intrinsically Disordered Proteins.J Biol Chem. 2016 Mar 25;291(13):6681-8. doi: 10.1074/jbc.R115.685859. Epub 2016 Feb 5. J Biol Chem. 2016. PMID: 26851286 Free PMC article. Review.
-
Structural features and interfacial properties of WH2, β-thymosin domains and other intrinsically disordered domains in the regulation of actin cytoskeleton dynamics.Cytoskeleton (Hoboken). 2013 Nov;70(11):686-705. doi: 10.1002/cm.21140. Epub 2013 Oct 22. Cytoskeleton (Hoboken). 2013. PMID: 24027208 Review.
-
Characterization of intrinsically disordered proteins and their dynamic complexes: From in vitro to cell-like environments.Prog Nucl Magn Reson Spectrosc. 2018 Dec;109:79-100. doi: 10.1016/j.pnmrs.2018.07.001. Epub 2018 Jul 31. Prog Nucl Magn Reson Spectrosc. 2018. PMID: 30527137 Review.
-
Structural biology of intrinsically disordered proteins: Revisiting unsolved mysteries.Biochimie. 2016 Jun;125:112-8. doi: 10.1016/j.biochi.2016.03.006. Epub 2016 Mar 19. Biochimie. 2016. PMID: 27004461 Review.
-
The multifaceted roles of intrinsic disorder in protein complexes.FEBS Lett. 2015 Sep 14;589(19 Pt A):2498-506. doi: 10.1016/j.febslet.2015.06.004. Epub 2015 Jun 11. FEBS Lett. 2015. PMID: 26073257 Review.
Cited by
-
Phase separation of TPX2 enhances and spatially coordinates microtubule nucleation.Nat Commun. 2020 Jan 14;11(1):270. doi: 10.1038/s41467-019-14087-0. Nat Commun. 2020. PMID: 31937751 Free PMC article.
-
Diffusion of a disordered protein on its folded ligand.Proc Natl Acad Sci U S A. 2021 Sep 14;118(37):e2106690118. doi: 10.1073/pnas.2106690118. Proc Natl Acad Sci U S A. 2021. PMID: 34504002 Free PMC article.
-
Structural basis for ELL2 and AFF4 activation of HIV-1 proviral transcription.Nat Commun. 2017 Jan 30;8:14076. doi: 10.1038/ncomms14076. Nat Commun. 2017. PMID: 28134250 Free PMC article.
-
Small-molecule sequestration of amyloid-β as a drug discovery strategy for Alzheimer's disease.Sci Adv. 2020 Nov 4;6(45):eabb5924. doi: 10.1126/sciadv.abb5924. Print 2020 Nov. Sci Adv. 2020. PMID: 33148639 Free PMC article.
-
A cyclin D1 intrinsically disordered domain accesses modified histone motifs to govern gene transcription.Oncogenesis. 2024 Jan 8;13(1):4. doi: 10.1038/s41389-023-00502-1. Oncogenesis. 2024. PMID: 38191593 Free PMC article.
References
-
- Frauenfelder H, Sligar SG, Wolynes PG. The Energy Landscapes and Motions of Proteins. Science. 1991;254:1598–1603. - PubMed
-
- Biehl R, Richter D. Slow Internal Protein Dynamics in Solution. J. Phys. Condens. Matter. 2014;26:503103. - PubMed
-
- Tompa P. Intrinsically Disordered Proteins: A 10-Year Recap. Trends Biochem. Sci. 2012;37:509–516. - PubMed
-
- Cohen P. The Regulation of Protein Function by Multisite Phosphorylation--a 25 Year Update. Trends Biochem. Sci. 2000;25:596–601. - PubMed
Publication types
MeSH terms
Substances
Grants and funding
LinkOut - more resources
Full Text Sources
Other Literature Sources