Imaging-guided delivery of RNAi for anticancer treatment
- PMID: 26805788
- PMCID: PMC5226392
- DOI: 10.1016/j.addr.2016.01.008
Imaging-guided delivery of RNAi for anticancer treatment
Abstract
The RNA interference (RNAi) technique is a new modality for cancer therapy, and several candidates are being tested clinically. In the development of RNAi-based therapeutics, imaging methods can provide a visible and quantitative way to investigate the therapeutic effect at anatomical, cellular, and molecular level; to noninvasively trace the distribution; to and study the biological processes in preclinical and clinical stages. Their abilities are important not only for therapeutic optimization and evaluation but also for shortening of the time of drug development to market. Typically, imaging-functionalized RNAi therapeutics delivery that combines nanovehicles and imaging techniques to study and improve their biodistribution and accumulation in tumor site has been progressively integrated into anticancer drug discovery and development processes. This review presents an overview of the current status of translating the RNAi cancer therapeutics in the clinic, a brief description of the biological barriers in drug delivery, and the roles of imaging in aspects of administration route, systemic circulation, and cellular barriers for the clinical translation of RNAi cancer therapeutics, and with partial content for discussing the safety concerns. Finally, we focus on imaging-guided delivery of RNAi therapeutics in preclinical development, including the basic principles of different imaging modalities, and their advantages and limitations for biological imaging. With growing number of RNAi therapeutics entering the clinic, various imaging methods will play an important role in facilitating the translation of RNAi cancer therapeutics from bench to bedside.
Keywords: Cancer; Gene delivery; Molecular imaging; Nanocarrier; RNA interference; Theranostics; siRNA.
Published by Elsevier B.V.
Figures
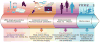
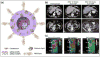
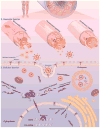
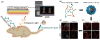
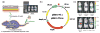
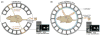
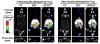
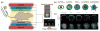
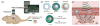
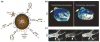
Similar articles
-
Development of RNAi technology for targeted therapy--a track of siRNA based agents to RNAi therapeutics.J Control Release. 2014 Nov 10;193:270-81. doi: 10.1016/j.jconrel.2014.04.044. Epub 2014 May 6. J Control Release. 2014. PMID: 24816071 Review.
-
Delivery and biodistribution of siRNA for cancer therapy: challenges and future prospects.Ther Deliv. 2012 Feb;3(2):245-61. doi: 10.4155/tde.11.155. Ther Deliv. 2012. PMID: 22834200 Review.
-
In vivo application of RNA interference: from functional genomics to therapeutics.Adv Genet. 2005;54:117-42. doi: 10.1016/S0065-2660(05)54006-9. Adv Genet. 2005. PMID: 16096010 Free PMC article. Review.
-
Current issues of RNAi therapeutics delivery and development.J Control Release. 2014 Dec 10;195:49-54. doi: 10.1016/j.jconrel.2014.07.056. Epub 2014 Aug 9. J Control Release. 2014. PMID: 25111131 Review.
-
Nano-based delivery of RNAi in cancer therapy.Mol Cancer. 2017 Jul 28;16(1):134. doi: 10.1186/s12943-017-0683-y. Mol Cancer. 2017. PMID: 28754120 Free PMC article. Review.
Cited by
-
Stimuli-responsive nanocarriers for drug delivery, tumor imaging, therapy and theranostics.Theranostics. 2020 Mar 15;10(10):4557-4588. doi: 10.7150/thno.38069. eCollection 2020. Theranostics. 2020. PMID: 32292515 Free PMC article. Review.
-
Inorganic Nanocarriers Overcoming Multidrug Resistance for Cancer Theranostics.Adv Sci (Weinh). 2016 May 30;3(11):1600134. doi: 10.1002/advs.201600134. eCollection 2016 Nov. Adv Sci (Weinh). 2016. PMID: 27980988 Free PMC article.
-
Graphene-based nanomaterials for bioimaging.Adv Drug Deliv Rev. 2016 Oct 1;105(Pt B):242-254. doi: 10.1016/j.addr.2016.05.013. Epub 2016 May 24. Adv Drug Deliv Rev. 2016. PMID: 27233213 Free PMC article. Review.
-
Exploration of Site-Specific Drug Targeting-A Review on EPR-, Stimuli-, Chemical-, and Receptor-Based Approaches as Potential Drug Targeting Methods in Cancer Treatment.J Oncol. 2022 Sep 29;2022:9396760. doi: 10.1155/2022/9396760. eCollection 2022. J Oncol. 2022. PMID: 36284633 Free PMC article. Review.
-
Exploiting Nanomaterial-mediated Autophagy for Cancer Therapy.Small Methods. 2019 Feb 13;3(2):1800365. doi: 10.1002/smtd.201800365. Epub 2018 Nov 15. Small Methods. 2019. PMID: 31355327 Free PMC article.
References
-
- Fire A, Xu S, Montgomery MK, Kostas SA, Driver SE, Mello CC. Potent and specific genetic interference by double-stranded RNA in Caenorhabditis elegans. Nature. 1998;391:806–811. - PubMed
-
- Elbashir SM, Harborth J, Lendeckel W, Yalcin A, Weber K, Tuschl T. Duplexes of 21-nucleotide RNAs mediate RNA interference in cultured mammalian cells. Nature. 2001;411:494–498. - PubMed
-
- Ginn SL, Alexander IE, Edelstein ML, Abedi MR, Wixon J. Gene therapy clinical trials worldwide to 2012 -an update. J Gene Med. 2013;15:65–77. - PubMed
-
- Kaiser PK, Symons RC, Shah SM, Quinlan EJ, Tabandeh H, Do DV, Reisen G, Lockridge JA, Short B, Guerciolini R, Nguyen QD. RNAi-based treatment for neovascular age-related macular degeneration by Sirna-027. Am J Ophthalmol. 2010;150:33–39.e32. - PubMed
Publication types
MeSH terms
Substances
Grants and funding
LinkOut - more resources
Full Text Sources
Other Literature Sources
Medical