An Outer Mitochondrial Translocase, Tom22, Is Crucial for Inner Mitochondrial Steroidogenic Regulation in Adrenal and Gonadal Tissues
- PMID: 26787839
- PMCID: PMC4810470
- DOI: 10.1128/MCB.01107-15
An Outer Mitochondrial Translocase, Tom22, Is Crucial for Inner Mitochondrial Steroidogenic Regulation in Adrenal and Gonadal Tissues
Abstract
After cholesterol is transported into the mitochondria of steroidogenic tissues, the first steroid, pregnenolone, is synthesized in adrenal and gonadal tissues to initiate steroid synthesis by catalyzing the conversion of pregnenolone to progesterone, which is mediated by the inner mitochondrial enzyme 3β-hydroxysteroid dehydrogenase 2 (3βHSD2). We report that the mitochondrial translocase Tom22 is essential for metabolic conversion, as its knockdown by small interfering RNA (siRNA) completely ablated progesterone conversion in both steroidogenic mouse Leydig MA-10 and human adrenal NCI cells. Tom22 forms a 500-kDa complex with mitochondrial proteins associated with 3βHSD2. Although the absence of Tom22 did not inhibit mitochondrial import of cytochrome P450scc (cytochrome P450 side chain cleavage enzyme) and aldosterone synthase, it did inhibit 3βHSD2 expression. Electron microscopy showed that Tom22 is localized at the outer mitochondrial membrane (OMM), while 3βHSD2 is localized at the inner mitochondrial space (IMS), where it interacts through a specific region with Tom22 with its C-terminal amino acids and a small amino acid segment of Tom22 exposed to the IMS. Therefore, Tom22 is a critical regulator of steroidogenesis, and thus, it is essential for mammalian survival.
Copyright © 2016, American Society for Microbiology. All Rights Reserved.
Figures
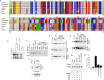
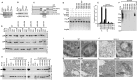
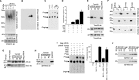
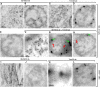
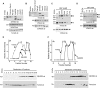
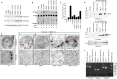
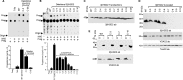
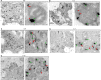
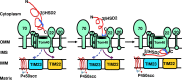
Similar articles
-
Inner mitochondrial translocase Tim50 interacts with 3β-hydroxysteroid dehydrogenase type 2 to regulate adrenal and gonadal steroidogenesis.J Biol Chem. 2011 Nov 11;286(45):39130-40. doi: 10.1074/jbc.M111.290031. Epub 2011 Sep 19. J Biol Chem. 2011. PMID: 21930695 Free PMC article.
-
Steroid hormone synthesis in mitochondria.Mol Cell Endocrinol. 2013 Oct 15;379(1-2):62-73. doi: 10.1016/j.mce.2013.04.014. Epub 2013 Apr 28. Mol Cell Endocrinol. 2013. PMID: 23628605
-
Lipid-mediated unfolding of 3β-hydroxysteroid dehydrogenase 2 is essential for steroidogenic activity.Biochemistry. 2011 Dec 27;50(51):11015-24. doi: 10.1021/bi2016102. Epub 2011 Dec 6. Biochemistry. 2011. PMID: 22106846 Free PMC article.
-
Regulation of human 3-beta-hydroxysteroid dehydrogenase type-2 (3βHSD2) by molecular chaperones and the mitochondrial environment affects steroidogenesis.J Steroid Biochem Mol Biol. 2015 Jul;151:74-84. doi: 10.1016/j.jsbmb.2014.11.018. Epub 2014 Nov 21. J Steroid Biochem Mol Biol. 2015. PMID: 25448736 Review.
-
Hormonal regulation of steroidogenic enzyme gene expression in Leydig cells.J Steroid Biochem Mol Biol. 1992 Dec;43(8):895-906. doi: 10.1016/0960-0760(92)90317-C. J Steroid Biochem Mol Biol. 1992. PMID: 22217834 Review.
Cited by
-
46,XX DSD due to Androgen Excess in Monogenic Disorders of Steroidogenesis: Genetic, Biochemical, and Clinical Features.Int J Mol Sci. 2019 Sep 17;20(18):4605. doi: 10.3390/ijms20184605. Int J Mol Sci. 2019. PMID: 31533357 Free PMC article. Review.
-
A single cell level measurement of StAR expression and activity in adrenal cells.Mol Cell Endocrinol. 2017 Feb 5;441:22-30. doi: 10.1016/j.mce.2016.08.015. Epub 2016 Aug 10. Mol Cell Endocrinol. 2017. PMID: 27521960 Free PMC article. Review.
-
Maternal betaine suppresses adrenal expression of cholesterol trafficking genes and decreases plasma corticosterone concentration in offspring pullets.J Anim Sci Biotechnol. 2019 Nov 19;10:87. doi: 10.1186/s40104-019-0396-8. eCollection 2019. J Anim Sci Biotechnol. 2019. PMID: 31827786 Free PMC article.
-
Mitochondrial metabolic regulation by GRP78.Sci Adv. 2017 Feb 24;3(2):e1602038. doi: 10.1126/sciadv.1602038. eCollection 2017 Feb. Sci Adv. 2017. PMID: 28275724 Free PMC article.
-
Tom40 in cholesterol transport.iScience. 2023 Mar 11;26(4):106386. doi: 10.1016/j.isci.2023.106386. eCollection 2023 Apr 21. iScience. 2023. PMID: 37035007 Free PMC article.
References
-
- Glick BS, Brandt A, Cunningham K, Muller S, Hallberg RL, Schatz G. 1992. Cytochrome c1 and b2 are sorted to the intermembrane space of yeast mitochondria by a stop-transfer mechanism. Cell 69:347–357. - PubMed
Publication types
MeSH terms
Substances
Grants and funding
LinkOut - more resources
Full Text Sources
Other Literature Sources
Molecular Biology Databases