Skeletal Mineralization Deficits and Impaired Biogenesis and Function of Chondrocyte-Derived Matrix Vesicles in Phospho1(-/-) and Phospho1/Pi t1 Double-Knockout Mice
- PMID: 26773408
- PMCID: PMC4891278
- DOI: 10.1002/jbmr.2790
Skeletal Mineralization Deficits and Impaired Biogenesis and Function of Chondrocyte-Derived Matrix Vesicles in Phospho1(-/-) and Phospho1/Pi t1 Double-Knockout Mice
Abstract
We have previously shown that ablation of either the Phospho1 or Alpl gene, encoding PHOSPHO1 and tissue-nonspecific alkaline phosphatase (TNAP) respectively, lead to hyperosteoidosis, but that their chondrocyte-derived and osteoblast-derived matrix vesicles (MVs) are able to initiate mineralization. In contrast, the double ablation of Phospho1 and Alpl completely abolish initiation and progression of skeletal mineralization. We argued that MVs initiate mineralization by a dual mechanism: PHOSPHO1-mediated intravesicular generation of inorganic phosphate (Pi ) and phosphate transporter-mediated influx of Pi . To test this hypothesis, we generated mice with col2a1-driven Cre-mediated ablation of Slc20a1, hereafter referred to as Pi t1, alone or in combination with a Phospho1 gene deletion. Pi t1(col2/col2) mice did not show any major phenotypic abnormalities, whereas severe skeletal deformities were observed in the [Phospho1(-/-) ; Pi t1(col2/col2) ] double knockout mice that were more pronounced than those observed in the Phospho1(-/-) mice. Histological analysis of [Phospho1(-/-) ; Pi t1(col2/col2) ] bones showed growth plate abnormalities with a shorter hypertrophic chondrocyte zone and extensive hyperosteoidosis. The [Phospho1(-/-) ; Pi t1(col2/col2) ] skeleton displayed significant decreases in BV/TV%, trabecular number, and bone mineral density, as well as decreased stiffness, decreased strength, and increased postyield deflection compared to Phospho1(-/-) mice. Using atomic force microscopy we found that ∼80% of [Phospho1(-/-) ; Pi t1(col2/col2) ] MVs were devoid of mineral in comparison to ∼50% for the Phospho1(-/-) MVs and ∼25% for the WT and Pi t1(col2/col2) MVs. We also found a significant decrease in the number of MVs produced by both Phospho1(-/-) and [Phospho1(-/-) ; Pi t1(col2/col2) ] chondrocytes. These data support the involvement of phosphate transporter 1, hereafter referred to as Pi T-1, in the initiation of skeletal mineralization and provide compelling evidence that PHOSPHO1 function is involved in MV biogenesis. © 2016 American Society for Bone and Mineral Research.
Keywords: DEVELOPMENT; GENETIC ANIMAL MODELS; GROWTH PLATE; MOLECULAR PATHWAYS.
© 2016 American Society for Bone and Mineral Research.
Conflict of interest statement
Conflict of interest: All authors report no conflicts of interest.
Figures
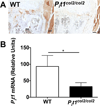
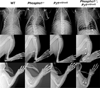
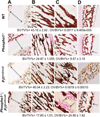
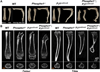
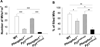
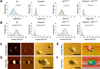
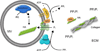
Similar articles
-
The role of phosphatases in the initiation of skeletal mineralization.Calcif Tissue Int. 2013 Oct;93(4):299-306. doi: 10.1007/s00223-012-9672-8. Epub 2012 Nov 27. Calcif Tissue Int. 2013. PMID: 23183786 Free PMC article. Review.
-
Loss of skeletal mineralization by the simultaneous ablation of PHOSPHO1 and alkaline phosphatase function: a unified model of the mechanisms of initiation of skeletal calcification.J Bone Miner Res. 2011 Feb;26(2):286-97. doi: 10.1002/jbmr.195. Epub 2010 Aug 3. J Bone Miner Res. 2011. PMID: 20684022 Free PMC article.
-
Functional involvement of PHOSPHO1 in matrix vesicle-mediated skeletal mineralization.J Bone Miner Res. 2007 Apr;22(4):617-27. doi: 10.1359/jbmr.070108. J Bone Miner Res. 2007. PMID: 17227223
-
Inhibition of PHOSPHO1 activity results in impaired skeletal mineralization during limb development of the chick.Bone. 2010 Apr;46(4):1146-55. doi: 10.1016/j.bone.2009.12.018. Epub 2010 Jan 4. Bone. 2010. PMID: 20053388 Free PMC article.
-
PLA2 and ENPP6 may act in concert to generate phosphocholine from the matrix vesicle membrane during skeletal mineralization.FASEB J. 2018 Jan;32(1):20-25. doi: 10.1096/fj.201700521R. Epub 2017 Sep 1. FASEB J. 2018. PMID: 28864658 Review.
Cited by
-
Gene Therapy Using Recombinant AAV Type 8 Vector Encoding TNAP-D10 Improves the Skeletal Phenotypes in Murine Models of Osteomalacia.JBMR Plus. 2022 Dec 15;7(1):e10709. doi: 10.1002/jbm4.10709. eCollection 2023 Jan. JBMR Plus. 2022. PMID: 36699639 Free PMC article.
-
Transgenic mouse model for conditional expression of influenza hemagglutinin-tagged human SLC20A1/PIT1.PLoS One. 2019 Oct 15;14(10):e0223052. doi: 10.1371/journal.pone.0223052. eCollection 2019. PLoS One. 2019. PMID: 31613887 Free PMC article.
-
Rickets in Children: An Update.Biomedicines. 2021 Jun 27;9(7):738. doi: 10.3390/biomedicines9070738. Biomedicines. 2021. PMID: 34199067 Free PMC article. Review.
-
Vascular calcification: from the perspective of crosstalk.Mol Biomed. 2023 Oct 18;4(1):35. doi: 10.1186/s43556-023-00146-y. Mol Biomed. 2023. PMID: 37851172 Free PMC article. Review.
-
Lipid composition modulates ATP hydrolysis and calcium phosphate mineral propagation by TNAP-harboring proteoliposomes.Arch Biochem Biophys. 2020 Sep 30;691:108482. doi: 10.1016/j.abb.2020.108482. Epub 2020 Jul 22. Arch Biochem Biophys. 2020. PMID: 32710882 Free PMC article.
References
-
- Majeska RJ, Wuthier RE. Studies on matrix vesicles isolated from chick epiphyseal cartilage. Association of pyrophosphatase and ATPase activities with alkaline phosphatase. Biochim Biophys Acta. 1975;391(1):51–60. - PubMed
-
- Johnson KA, Hessle L, Vaingankar S, et al. Osteoblast tissue-nonspecific alkaline phosphatase antagonizes and regulates PC-1. Am J Physiol Regul Integr Comp Physiol. 2000;279(4):R1365–R1377. - PubMed
Publication types
MeSH terms
Substances
Grants and funding
LinkOut - more resources
Full Text Sources
Other Literature Sources
Medical
Molecular Biology Databases
Research Materials
Miscellaneous