The Emerging Hallmarks of Cancer Metabolism
- PMID: 26771115
- PMCID: PMC4715268
- DOI: 10.1016/j.cmet.2015.12.006
The Emerging Hallmarks of Cancer Metabolism
Abstract
Tumorigenesis is dependent on the reprogramming of cellular metabolism as both direct and indirect consequence of oncogenic mutations. A common feature of cancer cell metabolism is the ability to acquire necessary nutrients from a frequently nutrient-poor environment and utilize these nutrients to both maintain viability and build new biomass. The alterations in intracellular and extracellular metabolites that can accompany cancer-associated metabolic reprogramming have profound effects on gene expression, cellular differentiation, and the tumor microenvironment. In this Perspective, we have organized known cancer-associated metabolic changes into six hallmarks: (1) deregulated uptake of glucose and amino acids, (2) use of opportunistic modes of nutrient acquisition, (3) use of glycolysis/TCA cycle intermediates for biosynthesis and NADPH production, (4) increased demand for nitrogen, (5) alterations in metabolite-driven gene regulation, and (6) metabolic interactions with the microenvironment. While few tumors display all six hallmarks, most display several. The specific hallmarks exhibited by an individual tumor may ultimately contribute to better tumor classification and aid in directing treatment.
Copyright © 2016 Elsevier Inc. All rights reserved.
Figures
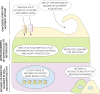
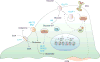
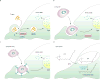
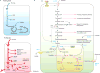
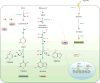
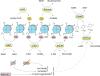
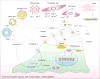
Similar articles
-
Metabolic reprogramming: the emerging concept and associated therapeutic strategies.J Exp Clin Cancer Res. 2015 Oct 6;34:111. doi: 10.1186/s13046-015-0221-y. J Exp Clin Cancer Res. 2015. PMID: 26445347 Free PMC article. Review.
-
Alternative fuels for cancer cells.Cancer J. 2015 Mar-Apr;21(2):49-55. doi: 10.1097/PPO.0000000000000104. Cancer J. 2015. PMID: 25815843 Free PMC article. Review.
-
Metabolic reprogramming in cancer: unraveling the role of glutamine in tumorigenesis.Semin Cell Dev Biol. 2012 Jun;23(4):362-9. doi: 10.1016/j.semcdb.2012.02.002. Epub 2012 Feb 11. Semin Cell Dev Biol. 2012. PMID: 22349059 Review.
-
Heterogeneity in Cancer Metabolism: New Concepts in an Old Field.Antioxid Redox Signal. 2017 Mar 20;26(9):462-485. doi: 10.1089/ars.2016.6750. Epub 2016 Jul 13. Antioxid Redox Signal. 2017. PMID: 27228792 Free PMC article. Review.
-
Antagonistic role of natural compounds in mTOR-mediated metabolic reprogramming.Cancer Lett. 2015 Jan 28;356(2 Pt A):251-62. doi: 10.1016/j.canlet.2014.02.008. Epub 2014 Feb 14. Cancer Lett. 2015. PMID: 24530513 Review.
Cited by
-
Methionine restriction breaks obligatory coupling of cell proliferation and death by an oncogene Src in Drosophila.Elife. 2021 Apr 27;10:e59809. doi: 10.7554/eLife.59809. Elife. 2021. PMID: 33902813 Free PMC article.
-
Metabolic reprogramming in renal cancer: Events of a metabolic disease.Biochim Biophys Acta Rev Cancer. 2021 Aug;1876(1):188559. doi: 10.1016/j.bbcan.2021.188559. Epub 2021 May 6. Biochim Biophys Acta Rev Cancer. 2021. PMID: 33965513 Free PMC article. Review.
-
Cancer-associated fibroblast-specific lncRNA LINC01614 enhances glutamine uptake in lung adenocarcinoma.J Hematol Oncol. 2022 Oct 8;15(1):141. doi: 10.1186/s13045-022-01359-4. J Hematol Oncol. 2022. PMID: 36209111 Free PMC article.
-
PER1 suppresses glycolysis and cell proliferation in oral squamous cell carcinoma via the PER1/RACK1/PI3K signaling complex.Cell Death Dis. 2021 Mar 15;12(3):276. doi: 10.1038/s41419-021-03563-5. Cell Death Dis. 2021. PMID: 33723221 Free PMC article.
-
Advances in sex disparities for cancer immunotherapy: unveiling the dilemma of Yin and Yang.Biol Sex Differ. 2022 Oct 22;13(1):58. doi: 10.1186/s13293-022-00469-5. Biol Sex Differ. 2022. PMID: 36273184 Free PMC article. Review.
References
-
- Balss J, Meyer J, Mueller W, Korshunov A, Hartmann C, von Deimling A. Analysis of the IDH1 codon 132 mutation in brain tumors. Acta Neuropathol. 2008;116:597–602. - PubMed
-
- Barthel A, Okino ST, Liao J, Nakatani K, Li J, Whitlock JP, Jr, Roth RA. Regulation of GLUT1 gene transcription by the serine/threonine kinase Akt1. J Biol Chem. 1999;274:20281–20286. - PubMed
-
- Bauer DE, Hatzivassiliou G, Zhao F, Andreadis C, Thompson CB. ATP citrate lyase is an important component of cell growth and transformation. Oncogene. 2005;24:6314–6322. - PubMed
Publication types
MeSH terms
Substances
Grants and funding
LinkOut - more resources
Full Text Sources
Other Literature Sources
Molecular Biology Databases