Imaging of the cross-presenting dendritic cell subsets in the skin-draining lymph node
- PMID: 26755602
- PMCID: PMC4743831
- DOI: 10.1073/pnas.1513607113
Imaging of the cross-presenting dendritic cell subsets in the skin-draining lymph node
Abstract
Dendritic cells (DCs) are antigen-presenting cells specialized for activating T cells to elicit effector T-cell functions. Cross-presenting DCs are a DC subset capable of presenting antigens to CD8(+) T cells and play critical roles in cytotoxic T-cell-mediated immune responses to microorganisms and cancer. Although their importance is known, the spatiotemporal dynamics of cross-presenting DCs in vivo are incompletely understood. Here, we study the T-cell zone in skin-draining lymph nodes (SDLNs) and find it is compartmentalized into regions for CD8(+) T-cell activation by cross-presenting DCs that express the chemokine (C motif) receptor 1 gene, Xcr1 and for CD4(+) T-cell activation by CD11b(+) DCs. Xcr1-expressing DCs in the SDLNs are composed of two different populations: migratory (CD103(hi)) DCs, which immigrate from the skin, and resident (CD8α(hi)) DCs, which develop in the nodes. To characterize the dynamic interactions of these distinct DC populations with CD8(+) T cells during their activation in vivo, we developed a photoconvertible reporter mouse strain, which permits us to distinctively visualize the migratory and resident subsets of Xcr1-expressing DCs. After leaving the skin, migratory DCs infiltrated to the deep T-cell zone of the SDLNs over 3 d, which corresponded to their half-life in the SDLNs. Intravital two-photon imaging showed that after soluble antigen immunization, the newly arriving migratory DCs more efficiently form sustained conjugates with antigen-specific CD8(+) T cells than other Xcr1-expressing DCs in the SDLNs. These results offer in vivo evidence for differential contributions of migratory and resident cross-presenting DCs to CD8(+) T-cell activation.
Keywords: CD8+ T cell; cross-presentation; dendritic cell; intravital two-photon imaging; photoconversion.
Conflict of interest statement
The authors declare no conflict of interest.
Figures
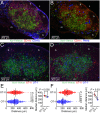
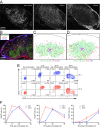
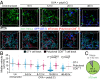
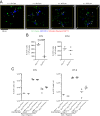
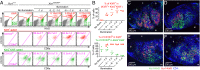
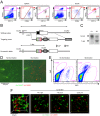
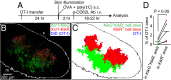
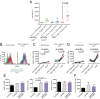
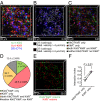
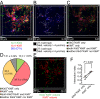
Similar articles
-
Spatiotemporally Distinct Interactions with Dendritic Cell Subsets Facilitates CD4+ and CD8+ T Cell Activation to Localized Viral Infection.Immunity. 2015 Sep 15;43(3):554-65. doi: 10.1016/j.immuni.2015.07.020. Epub 2015 Aug 18. Immunity. 2015. PMID: 26297566
-
A Highly Active Form of XCL1/Lymphotactin Functions as an Effective Adjuvant to Recruit Cross-Presenting Dendritic Cells for Induction of Effector and Memory CD8+ T Cells.Front Immunol. 2018 Nov 27;9:2775. doi: 10.3389/fimmu.2018.02775. eCollection 2018. Front Immunol. 2018. PMID: 30542351 Free PMC article.
-
CD103- and CD103+ bronchial lymph node dendritic cells are specialized in presenting and cross-presenting innocuous antigen to CD4+ and CD8+ T cells.J Immunol. 2007 Jun 1;178(11):6861-6. doi: 10.4049/jimmunol.178.11.6861. J Immunol. 2007. PMID: 17513734
-
Unique functions of splenic CD8alpha+ dendritic cells during infection with intracellular pathogens.Immunol Lett. 2007 Dec 15;114(2):66-72. doi: 10.1016/j.imlet.2007.09.007. Epub 2007 Oct 12. Immunol Lett. 2007. PMID: 17964665 Review.
-
Migratory conventional dendritic cells in the induction of peripheral T cell tolerance.J Leukoc Biol. 2013 Nov;94(5):903-11. doi: 10.1189/jlb.0413222. Epub 2013 Jul 29. J Leukoc Biol. 2013. PMID: 23898045 Review.
Cited by
-
Effects of VEGF blockade on the dynamics of the inflammatory landscape in glioblastoma-bearing mice.J Neuroinflammation. 2019 Oct 28;16(1):191. doi: 10.1186/s12974-019-1563-8. J Neuroinflammation. 2019. PMID: 31660979 Free PMC article.
-
CD8+ T Cells Orchestrate pDC-XCR1+ Dendritic Cell Spatial and Functional Cooperativity to Optimize Priming.Immunity. 2017 Feb 21;46(2):205-219. doi: 10.1016/j.immuni.2017.01.003. Epub 2017 Feb 9. Immunity. 2017. PMID: 28190711 Free PMC article.
-
Alarmins and immunity.Immunol Rev. 2017 Nov;280(1):41-56. doi: 10.1111/imr.12577. Immunol Rev. 2017. PMID: 29027222 Free PMC article. Review.
-
Brief exposure of skin to near-infrared laser augments early vaccine responses.Nanophotonics. 2021 Sep;10(12):3187-3197. doi: 10.1515/nanoph-2021-0133. Epub 2021 Aug 9. Nanophotonics. 2021. PMID: 34868804 Free PMC article.
-
Spatial Lymphocyte Dynamics in Lymph Nodes Predicts the Cytotoxic T Cell Frequency Needed for HIV Infection Control.Front Immunol. 2019 Jun 11;10:1213. doi: 10.3389/fimmu.2019.01213. eCollection 2019. Front Immunol. 2019. PMID: 31244829 Free PMC article.
References
-
- Mellman I, Steinman RM. Dendritic cells: Specialized and regulated antigen processing machines. Cell. 2001;106(3):255–258. - PubMed
-
- Henrickson SE, et al. In vivo imaging of T cell priming. Sci Signal. 2008;1(12):pt2. - PubMed
-
- Moreau HD, Bousso P. Visualizing how T cells collect activation signals in vivo. Curr Opin Immunol. 2014;26:56–62. - PubMed
-
- Joffre OP, Segura E, Savina A, Amigorena S. Cross-presentation by dendritic cells. Nat Rev Immunol. 2012;12(8):557–569. - PubMed
Publication types
MeSH terms
Substances
LinkOut - more resources
Full Text Sources
Other Literature Sources
Molecular Biology Databases
Research Materials