Rational design of Raman-labeled nanoparticles for a dual-modality, light scattering immunoassay on a polystyrene substrate
- PMID: 26751120
- PMCID: PMC4705623
- DOI: 10.1186/s13036-015-0023-y
Rational design of Raman-labeled nanoparticles for a dual-modality, light scattering immunoassay on a polystyrene substrate
Abstract
Background: Surface-enhanced Raman scattering (SERS) is a powerful light scattering technique that can be used for sensitive immunoassay development and cell labeling. A major obstacle to using SERS is the complexity of fabricating SERS probes since they require nanoscale characterization and optical uniformity. The light scattering response of SERS probes may also be modulated by the substrate used for SERS analysis. A typical SERS substrate such as quartz can be expensive. Polystyrene is a cheaper substrate option but can decrease the SERS response due to interfering Raman emission peaks and high background fluorescence. The goal of this research is to develop an optimized process for fabricating Raman-labeled nanoparticles for a SERS-based immunoassay on a polystyrene substrate.
Results: We have developed a method for fabricating SERS nanoparticle probes for use in a light scattering immunoassay on a polystyrene substrate. The light scattering profile of both spherical gold nanoparticle and gold nanorod SERS probes were characterized using Raman spectroscopy and optical absorbance spectroscopy. The effects of substrate interference and autofluorescence were reduced by selecting a Raman reporter with a strong light scattering response in a spectral region where interfering substrate emission peaks are minimized. Both spherical gold nanoparticles and gold nanorods SERS probes used in the immunoassay were detected at labeling concentrations in the low pM range. This analytical sensitivity falls within the typical dynamic range for direct labeling of cell-surface biomarkers using SERS probes.
Conclusion: SERS nanoparticle probes were fabricated to produce a strong light scattering signal despite substrate interference. The optical extinction and inelastic light scattering of these probes was detected by optical absorbance spectroscopy and Raman spectroscopy, respectively. This immunoassay demonstrates the feasibility of analyzing strongly enhanced Raman signals on polystyrene, which is an inexpensive yet non-ideal Raman substrate. The assay sensitivity, which is in the low pM range, suggests that these SERS probe particles could be used for Raman labeling of cell or tissue samples in a polystyrene tissue culture plate. With continued development, this approach could be used for direct labeling of multiple cell surface biomarkers on strongly interfering substrate platforms.
Keywords: High-throughput; Immunoassay; Multiplexing; Nanoparticle; Raman Spectroscopy; Surface-Enhanced Raman Spectroscopy/Scattering (SERS).
Figures
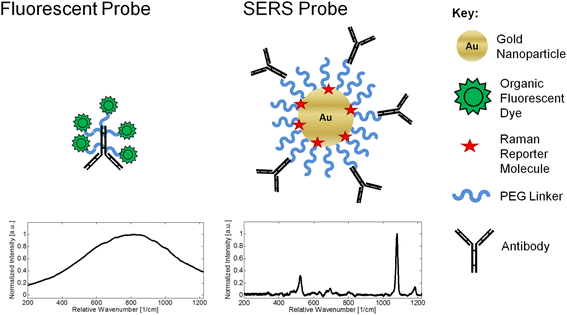
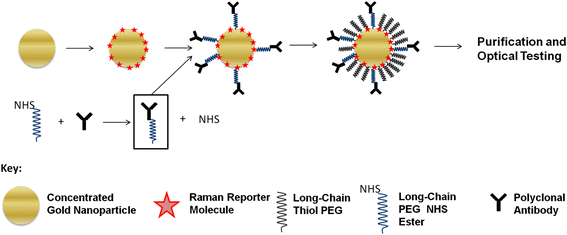
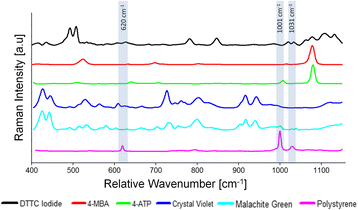
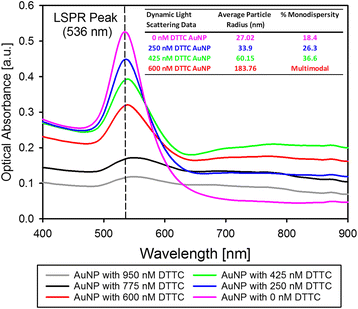
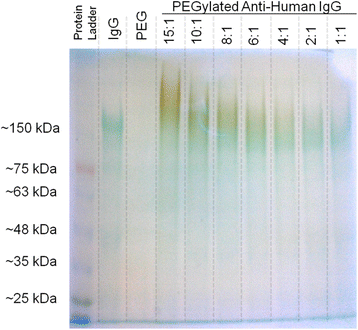
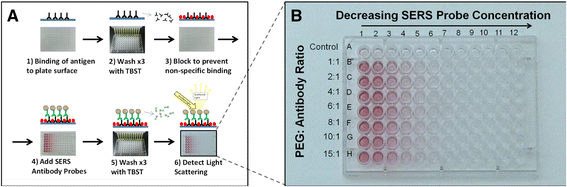
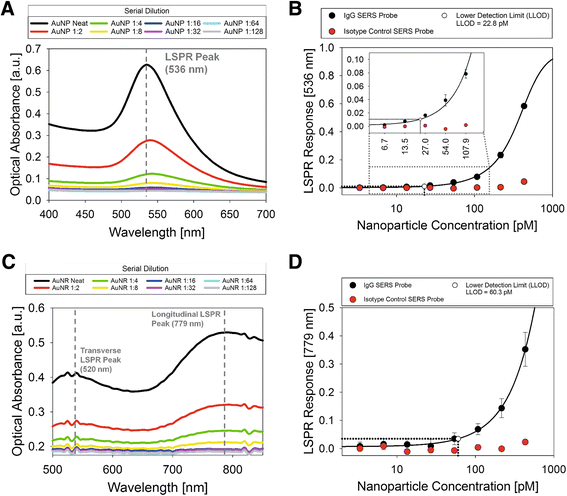
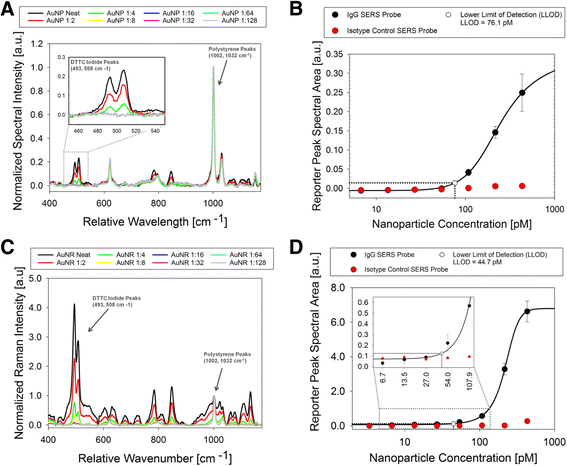
Similar articles
-
Highly sensitive immunoassay based on SERS using nano-Au immune probes and a nano-Ag immune substrate.Talanta. 2014 Jun;123:161-8. doi: 10.1016/j.talanta.2014.02.015. Epub 2014 Feb 14. Talanta. 2014. PMID: 24725879
-
Highly sensitive immunoassay based on Raman reporter-labeled immuno-Au aggregates and SERS-active immune substrate.Biosens Bioelectron. 2009 Dec 15;25(4):826-31. doi: 10.1016/j.bios.2009.08.035. Epub 2009 Aug 29. Biosens Bioelectron. 2009. PMID: 19765972
-
Gap-Dependent Surface-Enhanced Raman Scattering (SERS) Enhancement Model of SERS Substrate-Probe Combination Using a Polyelectrolyte Nanodroplet as a Distance Controller.Langmuir. 2021 Sep 14;37(36):10776-10785. doi: 10.1021/acs.langmuir.1c01556. Epub 2021 Aug 31. Langmuir. 2021. PMID: 34463518
-
Single cell analysis using surface enhanced Raman scattering (SERS) tags.Methods. 2012 Jul;57(3):272-9. doi: 10.1016/j.ymeth.2012.03.024. Epub 2012 Apr 4. Methods. 2012. PMID: 22498143 Free PMC article. Review.
-
Raman Scattering-Based Biosensing: New Prospects and Opportunities.Biosensors (Basel). 2021 Dec 13;11(12):512. doi: 10.3390/bios11120512. Biosensors (Basel). 2021. PMID: 34940269 Free PMC article. Review.
Cited by
-
Overview of the Design and Application of Dual-Signal Immunoassays.Molecules. 2024 Sep 25;29(19):4551. doi: 10.3390/molecules29194551. Molecules. 2024. PMID: 39407482 Free PMC article. Review.
-
Exploring Sensitive Label-Free Multiplex Analysis with Raman-Coded Microbeads and SERS-Coded Reporters.Biosensors (Basel). 2022 Feb 16;12(2):121. doi: 10.3390/bios12020121. Biosensors (Basel). 2022. PMID: 35200381 Free PMC article.
-
Fabricating a UV-Vis and Raman Spectroscopy Immunoassay Platform.J Vis Exp. 2016 Nov 10;(117):54795. doi: 10.3791/54795. J Vis Exp. 2016. PMID: 27911413 Free PMC article.
-
Lateral Flow Immunoassay of SARS-CoV-2 Antigen with SERS-Based Registration: Development and Comparison with Traditional Immunoassays.Biosensors (Basel). 2021 Dec 10;11(12):510. doi: 10.3390/bios11120510. Biosensors (Basel). 2021. PMID: 34940267 Free PMC article.
-
Alternative cDEP Design to Facilitate Cell Isolation for Identification by Raman Spectroscopy.Sensors (Basel). 2017 Feb 9;17(2):327. doi: 10.3390/s17020327. Sensors (Basel). 2017. PMID: 28208767 Free PMC article.
References
-
- Swerdlow SH. WHO Classification of Tumours of Haematopoietic and Lymphoid Tissues. 4. International Agency for Research on Cancer: Northwestern University; 2008.
LinkOut - more resources
Full Text Sources
Other Literature Sources
Miscellaneous