PKN3 is the major regulator of angiogenesis and tumor metastasis in mice
- PMID: 26742562
- PMCID: PMC4705536
- DOI: 10.1038/srep18979
PKN3 is the major regulator of angiogenesis and tumor metastasis in mice
Abstract
PKN, a conserved family member related to PKC, was the first protein kinase identified as a target of the small GTPase Rho. PKN is involved in various functions including cytoskeletal arrangement and cell adhesion. Furthermore, the enrichment of PKN3 mRNA in some cancer cell lines as well as its requirement in malignant prostate cell growth suggested its involvement in oncogenesis. Despite intensive research efforts, physiological as well as pathological roles of PKN3 in vivo remain elusive. Here, we generated mice with a targeted deletion of PKN3. The PKN3 knockout (KO) mice are viable and develop normally. However, the absence of PKN3 had an impact on angiogenesis as evidenced by marked suppressions of micro-vessel sprouting in ex vivo aortic ring assay and in vivo corneal pocket assay. Furthermore, the PKN3 KO mice exhibited an impaired lung metastasis of melanoma cells when administered from the tail vein. Importantly, PKN3 knock-down by small interfering RNA (siRNA) induced a glycosylation defect of cell-surface glycoproteins, including ICAM-1, integrin β1 and integrin α5 in HUVECs. Our data provide the first in vivo genetic demonstration that PKN3 plays critical roles in angiogenesis and tumor metastasis, and that defective maturation of cell surface glycoproteins might underlie these phenotypes.
Figures
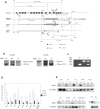
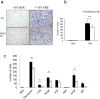
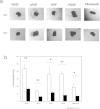
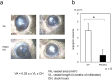
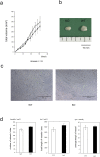
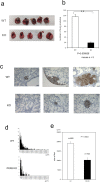
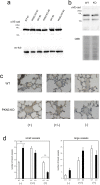
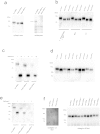
Similar articles
-
Targeted Disruption of JCAD (Junctional Protein Associated With Coronary Artery Disease)/KIAA1462, a Coronary Artery Disease-Associated Gene Product, Inhibits Angiogenic Processes In Vitro and In Vivo.Arterioscler Thromb Vasc Biol. 2017 Sep;37(9):1667-1673. doi: 10.1161/ATVBAHA.117.309721. Epub 2017 Jul 13. Arterioscler Thromb Vasc Biol. 2017. PMID: 28705794
-
Brag2 differentially regulates β1- and β3-integrin-dependent adhesion in endothelial cells and is involved in developmental and pathological angiogenesis.Basic Res Cardiol. 2014 Mar;109(2):404. doi: 10.1007/s00395-014-0404-2. Epub 2014 Feb 13. Basic Res Cardiol. 2014. PMID: 24522833
-
DUSP3/VHR is a pro-angiogenic atypical dual-specificity phosphatase.Mol Cancer. 2014 May 15;13:108. doi: 10.1186/1476-4598-13-108. Mol Cancer. 2014. PMID: 24886454 Free PMC article.
-
Pathological functions of the small GTPase Arf6 in cancer progression: Tumor angiogenesis and metastasis.Small GTPases. 2016 Apr 2;7(2):47-53. doi: 10.1080/21541248.2016.1154640. Epub 2016 Feb 24. Small GTPases. 2016. PMID: 26909552 Free PMC article. Review.
-
Efficient delivery of PKN3 shRNA for the treatment of breast cancer via lipid nanoparticles.Bioorg Med Chem. 2022 Sep 1;69:116884. doi: 10.1016/j.bmc.2022.116884. Epub 2022 Jun 13. Bioorg Med Chem. 2022. PMID: 35752145 Review.
Cited by
-
Identification of 4-Anilinoquin(az)oline as a Cell-Active Protein Kinase Novel 3 (PKN3) Inhibitor Chemotype.ChemMedChem. 2022 Jun 20;17(12):e202200161. doi: 10.1002/cmdc.202200161. Epub 2022 May 9. ChemMedChem. 2022. PMID: 35403825 Free PMC article.
-
Unraveling the intricacies of osteoclast differentiation and maturation: insight into novel therapeutic strategies for bone-destructive diseases.Exp Mol Med. 2024 Feb;56(2):264-272. doi: 10.1038/s12276-024-01157-7. Epub 2024 Feb 1. Exp Mol Med. 2024. PMID: 38297158 Free PMC article. Review.
-
Comparative Transcriptome Analysis of Spleen Reveals Potential Regulation of Genes and Immune Pathways Following Administration of Aeromonas salmonicida subsp. masoucida Vaccine in Atlantic Salmon (Salmo salar).Mar Biotechnol (NY). 2022 Mar;24(1):97-115. doi: 10.1007/s10126-021-10089-6. Epub 2022 Jan 27. Mar Biotechnol (NY). 2022. PMID: 35084599 Free PMC article.
-
PKN2 is involved in aggregation and spheroid formation of fibroblasts in suspension culture by regulating cell motility and N-cadherin expression.Biochem Biophys Rep. 2021 Jan 2;25:100895. doi: 10.1016/j.bbrep.2020.100895. eCollection 2021 Mar. Biochem Biophys Rep. 2021. PMID: 33437883 Free PMC article.
-
Protein kinase N1 critically regulates cerebellar development and long-term function.J Clin Invest. 2018 May 1;128(5):2076-2088. doi: 10.1172/JCI96165. Epub 2018 Apr 16. J Clin Invest. 2018. PMID: 29494346 Free PMC article.
References
-
- Mukai H. The structure and function of PKN, a protein kinase having a catalytic domain homologous to that of PKC. J Biochem. 133, 17–27 (2003). - PubMed
-
- Maesaki R. et al. The structural basis of Rho effector recognition revealed by the crystal structure of human RhoA complexed with the effector domain of PKN/PRK1. Mol Cell. 4, 793–803 (1999). - PubMed
-
- Oishi K., Mukai H., Shibata H., Takahashi M. & Ona Y. Identification and characterization of PKNbeta, a novel isoform of protein kinase PKN: expression and arachidonic acid dependency are different from those of PKNalpha. Biochem Biophys Res Commun. 261, 808–814 (1999). - PubMed
-
- Morrice N. A., Gabrielli B., Kemp B. E. & Wettenhall R. E. A cardiolipin-activated protein kinase from rat liver structurally distinct from the protein kinases C. J Biol Chem. 269, 20040–20046 (1994). - PubMed
-
- Mukai H. et al. Activation of PKN, a novel 120-kDa protein kinase with leucine zipper-like sequences, by unsaturated fatty acids and by limited proteolysis. Biochem Biophys Res Commun. 204, 348–356 (1994). - PubMed
Publication types
MeSH terms
Substances
LinkOut - more resources
Full Text Sources
Other Literature Sources
Molecular Biology Databases
Research Materials
Miscellaneous