Novel advances in shotgun lipidomics for biology and medicine
- PMID: 26703190
- PMCID: PMC4733395
- DOI: 10.1016/j.plipres.2015.12.002
Novel advances in shotgun lipidomics for biology and medicine
Abstract
The field of lipidomics, as coined in 2003, has made profound advances and been rapidly expanded. The mass spectrometry-based strategies of this analytical methodology-oriented research discipline for lipid analysis are largely fallen into three categories: direct infusion-based shotgun lipidomics, liquid chromatography-mass spectrometry-based platforms, and matrix-assisted laser desorption/ionization mass spectrometry-based approaches (particularly in imagining lipid distribution in tissues or cells). This review focuses on shotgun lipidomics. After briefly introducing its fundamentals, the major materials of this article cover its recent advances. These include the novel methods of lipid extraction, novel shotgun lipidomics strategies for identification and quantification of previously hardly accessible lipid classes and molecular species including isomers, and novel tools for processing and interpretation of lipidomics data. Representative applications of advanced shotgun lipidomics for biological and biomedical research are also presented in this review. We believe that with these novel advances in shotgun lipidomics, this approach for lipid analysis should become more comprehensive and high throughput, thereby greatly accelerating the lipidomics field to substantiate the aberrant lipid metabolism, signaling, trafficking, and homeostasis under pathological conditions and their underpinning biochemical mechanisms.
Keywords: Alzheimer's disease; Bioactive lipids; Lipidomics; Mass spectrometry; Metabolic syndrome; Shotgun lipidomics.
Copyright © 2015 Elsevier B.V. All rights reserved.
Figures
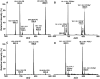
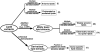
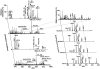
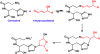
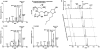

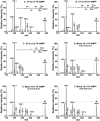
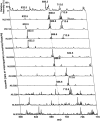
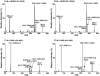
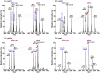
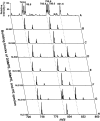
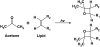
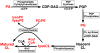
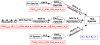
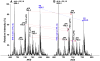
Similar articles
-
Strategies to Improve/Eliminate the Limitations in Shotgun Lipidomics.Proteomics. 2020 Jun;20(11):e1900070. doi: 10.1002/pmic.201900070. Epub 2019 Aug 8. Proteomics. 2020. PMID: 31291508 Free PMC article. Review.
-
Shotgun lipidomics in substantiating lipid peroxidation in redox biology: Methods and applications.Redox Biol. 2017 Aug;12:946-955. doi: 10.1016/j.redox.2017.04.030. Epub 2017 Apr 24. Redox Biol. 2017. PMID: 28494428 Free PMC article. Review.
-
Lipidomics: Techniques, Applications, and Outcomes Related to Biomedical Sciences.Trends Biochem Sci. 2016 Nov;41(11):954-969. doi: 10.1016/j.tibs.2016.08.010. Epub 2016 Sep 20. Trends Biochem Sci. 2016. PMID: 27663237 Free PMC article. Review.
-
Global Monitoring of the Mammalian Lipidome by Quantitative Shotgun Lipidomics.Methods Mol Biol. 2017;1609:123-139. doi: 10.1007/978-1-4939-6996-8_12. Methods Mol Biol. 2017. PMID: 28660579
-
Multi-dimensional mass spectrometry-based shotgun lipidomics and novel strategies for lipidomic analyses.Mass Spectrom Rev. 2012 Jan-Feb;31(1):134-78. doi: 10.1002/mas.20342. Epub 2011 Jul 13. Mass Spectrom Rev. 2012. PMID: 21755525 Free PMC article. Review.
Cited by
-
Monoacylglycerol Analysis Using MS/MS(ALL) Quadruple Time of Flight Mass Spectrometry.Metabolites. 2016 Aug 17;6(3):25. doi: 10.3390/metabo6030025. Metabolites. 2016. PMID: 27548241 Free PMC article.
-
Targeting DGAT1 Ameliorates Glioblastoma by Increasing Fat Catabolism and Oxidative Stress.Cell Metab. 2020 Aug 4;32(2):229-242.e8. doi: 10.1016/j.cmet.2020.06.002. Epub 2020 Jun 18. Cell Metab. 2020. PMID: 32559414 Free PMC article.
-
Analysis of monohexosyl alkyl (alkenyl)-acyl glycerol in brain samples by shotgun lipidomics.Anal Chim Acta. 2020 Sep 8;1129:143-149. doi: 10.1016/j.aca.2020.07.016. Epub 2020 Jul 24. Anal Chim Acta. 2020. PMID: 32891384 Free PMC article.
-
Quantitative Fragmentation Model for Bottom-Up Shotgun Lipidomics.Anal Chem. 2019 Sep 17;91(18):12085-12093. doi: 10.1021/acs.analchem.9b03270. Epub 2019 Sep 5. Anal Chem. 2019. PMID: 31441640 Free PMC article.
-
Proteomics and Lipidomics in Inflammatory Bowel Disease Research: From Mechanistic Insights to Biomarker Identification.Int J Mol Sci. 2018 Sep 15;19(9):2775. doi: 10.3390/ijms19092775. Int J Mol Sci. 2018. PMID: 30223557 Free PMC article. Review.
References
-
- Nomenclature I-ICoB. The nomenclature of lipids (recommendations 1976)
- Eur J Biochem. 1977;79:11–21. - PubMed
- Hoppe-Seylers Z Physiol Chem. 1977;358:617–631. - PubMed
- Lipids. 1977;12:455–468. - PubMed
- Mol Cell Biochem. 1977;17:157–171.
- Chem Phys Lipids. 1978;21:159–173.
- J Lipid Res. 1978;19:114–128. - PubMed
- Biochem J. 1978;171:21–35. http://www.chem.qmulac.uk/iupac/lipid/ - PMC - PubMed
-
- Fahy E, Subramaniam S, Brown HA, Glass CK, Merrill AH, Jr, Murphy RC, Raetz CR, Russell DW, Seyama Y, Shaw W, Shimizu T, Spener F, van Meer G, VanNieuwenhze MS, White SH, Witztum JL, Dennis EA. A comprehensive classification system for lipids. J Lipid Res. 2005;46:839–61. - PubMed
Publication types
MeSH terms
Substances
Grants and funding
LinkOut - more resources
Full Text Sources
Other Literature Sources