Structural Basis of Vesicle Formation at the Inner Nuclear Membrane
- PMID: 26687357
- PMCID: PMC4701712
- DOI: 10.1016/j.cell.2015.11.029
Structural Basis of Vesicle Formation at the Inner Nuclear Membrane
Abstract
Vesicular nucleo-cytoplasmic transport is becoming recognized as a general cellular mechanism for translocation of large cargoes across the nuclear envelope. Cargo is recruited, enveloped at the inner nuclear membrane (INM), and delivered by membrane fusion at the outer nuclear membrane. To understand the structural underpinning for this trafficking, we investigated nuclear egress of progeny herpesvirus capsids where capsid envelopment is mediated by two viral proteins, forming the nuclear egress complex (NEC). Using a multi-modal imaging approach, we visualized the NEC in situ forming coated vesicles of defined size. Cellular electron cryo-tomography revealed a protein layer showing two distinct hexagonal lattices at its membrane-proximal and membrane-distant faces, respectively. NEC coat architecture was determined by combining this information with integrative modeling using small-angle X-ray scattering data. The molecular arrangement of the NEC establishes the basic mechanism for budding and scission of tailored vesicles at the INM.
Copyright © 2015 The Authors. Published by Elsevier Inc. All rights reserved.
Figures
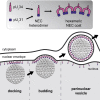
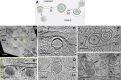
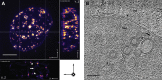
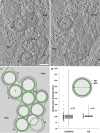
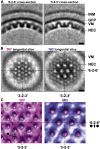
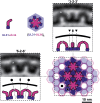
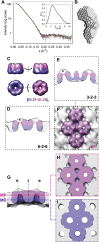
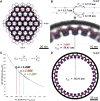
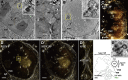
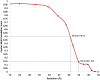
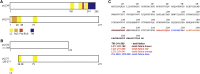
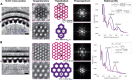
Similar articles
-
Mutational Functional Analysis of the Pseudorabies Virus Nuclear Egress Complex-Nucleocapsid Interaction.J Virol. 2020 Mar 31;94(8):e01910-19. doi: 10.1128/JVI.01910-19. Print 2020 Mar 31. J Virol. 2020. PMID: 32051272 Free PMC article.
-
Structural basis of membrane budding by the nuclear egress complex of herpesviruses.EMBO J. 2015 Dec 2;34(23):2921-36. doi: 10.15252/embj.201592359. Epub 2015 Oct 28. EMBO J. 2015. PMID: 26511020 Free PMC article.
-
The Primary Enveloped Virion of Herpes Simplex Virus 1: Its Role in Nuclear Egress.mBio. 2017 Jun 13;8(3):e00825-17. doi: 10.1128/mBio.00825-17. mBio. 2017. PMID: 28611252 Free PMC article.
-
The Knowns and Unknowns of Herpesvirus Nuclear Egress.Annu Rev Virol. 2023 Sep 29;10(1):305-323. doi: 10.1146/annurev-virology-111821-105518. Epub 2023 Apr 11. Annu Rev Virol. 2023. PMID: 37040797 Review.
-
Have NEC Coat, Will Travel: Structural Basis of Membrane Budding During Nuclear Egress in Herpesviruses.Adv Virus Res. 2017;97:107-141. doi: 10.1016/bs.aivir.2016.07.002. Epub 2016 Sep 1. Adv Virus Res. 2017. PMID: 28057257 Free PMC article. Review.
Cited by
-
Herpes Simplex Virus 1 Induces Phosphorylation and Reorganization of Lamin A/C through the γ134.5 Protein That Facilitates Nuclear Egress.J Virol. 2016 Oct 28;90(22):10414-10422. doi: 10.1128/JVI.01392-16. Print 2016 Nov 15. J Virol. 2016. PMID: 27630226 Free PMC article.
-
The universal suppressor mutation restores membrane budding defects in the HSV-1 nuclear egress complex by stabilizing the oligomeric lattice.PLoS Pathog. 2024 Jan 16;20(1):e1011936. doi: 10.1371/journal.ppat.1011936. eCollection 2024 Jan. PLoS Pathog. 2024. PMID: 38227586 Free PMC article.
-
HVint: A Strategy for Identifying Novel Protein-Protein Interactions in Herpes Simplex Virus Type 1.Mol Cell Proteomics. 2016 Sep;15(9):2939-53. doi: 10.1074/mcp.M116.058552. Epub 2016 Jul 6. Mol Cell Proteomics. 2016. PMID: 27384951 Free PMC article.
-
The viral replication organelles within cells studied by electron microscopy.Adv Virus Res. 2019;105:1-33. doi: 10.1016/bs.aivir.2019.07.005. Epub 2019 Aug 19. Adv Virus Res. 2019. PMID: 31522702 Free PMC article.
-
Nuclear envelope impairment is facilitated by the herpes simplex virus 1 Us3 kinase.F1000Res. 2019 Feb 18;8:198. doi: 10.12688/f1000research.17802.1. eCollection 2019. F1000Res. 2019. PMID: 31249678 Free PMC article.
References
Publication types
MeSH terms
Substances
Grants and funding
LinkOut - more resources
Full Text Sources
Other Literature Sources