Microvascular basis for growth of small infarcts following occlusion of single penetrating arterioles in mouse cortex
- PMID: 26661182
- PMCID: PMC4976746
- DOI: 10.1177/0271678X15608388
Microvascular basis for growth of small infarcts following occlusion of single penetrating arterioles in mouse cortex
Abstract
Small cerebral infarcts, i.e. microinfarcts, are common in the aging brain and linked to vascular cognitive impairment. However, little is known about the acute growth of these minute lesions and their effect on blood flow in surrounding tissues. We modeled microinfarcts in the mouse cortex by inducing photothrombotic clots in single penetrating arterioles. The resultant hemodynamic changes in tissues surrounding the occluded vessel were then studied using in vivo two-photon microscopy. We were able to generate a spectrum of infarct volumes by occluding arterioles that carried a range of blood fluxes. Those resulting from occlusion of high-flux penetrating arterioles (flux of 2 nL/s or higher) exhibited a radial outgrowth that encompassed unusually large tissue volumes. The gradual expansion of these infarcts was propagated by an evolving insufficiency in capillary flow that encroached on territories of neighboring penetrating arterioles, leading to the stagnation and recruitment of their perfusion domains into the final infarct volume. Our results suggest that local collapse of microvascular function contributes to tissue damage incurred by single penetrating arteriole occlusions in mice, and that a similar mechanism may add to pathophysiology induced by microinfarcts of the human brain.
Keywords: Ischemia; cerebral blood flow; microcirculation; two-photon microscopy; venous thrombosis.
© The Author(s) 2015.
Figures
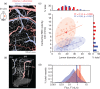
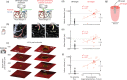
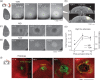
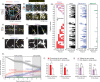
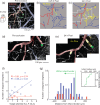
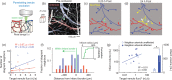
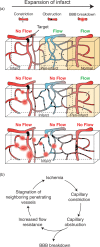
Similar articles
-
Penumbral microcirculatory changes associated with peri-infarct depolarizations in the rat.Stroke. 2002 Feb;33(2):606-12. doi: 10.1161/hs0202.102738. Stroke. 2002. PMID: 11823677
-
Collateral blood flow in different cerebrovascular hierarchy provides endogenous protection in cerebral ischemia.Brain Pathol. 2017 Nov;27(6):809-821. doi: 10.1111/bpa.12458. Epub 2017 Mar 27. Brain Pathol. 2017. PMID: 27859886 Free PMC article.
-
Robust and fragile aspects of cortical blood flow in relation to the underlying angioarchitecture.Microcirculation. 2015 Apr;22(3):204-218. doi: 10.1111/micc.12195. Microcirculation. 2015. PMID: 25705966 Free PMC article. Review.
-
Does pathology of small venules contribute to cerebral microinfarcts and dementia?J Neurochem. 2018 Mar;144(5):517-526. doi: 10.1111/jnc.14228. Epub 2017 Nov 7. J Neurochem. 2018. PMID: 28950410 Free PMC article. Review.
-
Functional deficits induced by cortical microinfarcts.J Cereb Blood Flow Metab. 2017 Nov;37(11):3599-3614. doi: 10.1177/0271678X16685573. Epub 2017 Jan 16. J Cereb Blood Flow Metab. 2017. PMID: 28090802 Free PMC article.
Cited by
-
Microvessel occlusions alter amyloid-beta plaque morphology in a mouse model of Alzheimer's disease.J Cereb Blood Flow Metab. 2020 Oct;40(10):2115-2131. doi: 10.1177/0271678X19889092. Epub 2019 Nov 19. J Cereb Blood Flow Metab. 2020. PMID: 31744388 Free PMC article.
-
Adhesion molecules and cerebral microvascular hemodynamic abnormalities in sickle cell disease.Front Neurol. 2022 Dec 7;13:976063. doi: 10.3389/fneur.2022.976063. eCollection 2022. Front Neurol. 2022. PMID: 36570439 Free PMC article.
-
Endothelial KIR2 channel dysfunction in aged cerebral parenchymal arterioles.Am J Physiol Heart Circ Physiol. 2023 Oct 6;325(6):H1360-72. doi: 10.1152/ajpheart.00279.2023. Online ahead of print. Am J Physiol Heart Circ Physiol. 2023. PMID: 37801044 Free PMC article.
-
Localizing Microemboli within the Rodent Brain through Block-Face Imaging and Atlas Registration.eNeuro. 2021 Aug 3;8(4):ENEURO.0216-21.2021. doi: 10.1523/ENEURO.0216-21.2021. Print 2021 Jul-Aug. eNeuro. 2021. PMID: 34272259 Free PMC article.
-
Pixel-by-pixel precise delay correction for measurement of cerebral hemodynamic parameters in H215O PET study.Ann Nucl Med. 2017 May;31(4):283-294. doi: 10.1007/s12149-017-1156-5. Epub 2017 Feb 27. Ann Nucl Med. 2017. PMID: 28243845 Free PMC article.
References
-
- Kövari E, Gold G, Herrmann FR, et al. Cortical microinfarcts and demyelination significantly affect cognition in brain aging. Stroke 2004; 35: 410–414. - PubMed
Publication types
MeSH terms
Grants and funding
LinkOut - more resources
Full Text Sources
Other Literature Sources