Comparative Circadian Metabolomics Reveal Differential Effects of Nutritional Challenge in the Serum and Liver
- PMID: 26644470
- PMCID: PMC4742746
- DOI: 10.1074/jbc.M115.681130
Comparative Circadian Metabolomics Reveal Differential Effects of Nutritional Challenge in the Serum and Liver
Abstract
Diagnosis and therapeutic interventions in pathological conditions rely upon clinical monitoring of key metabolites in the serum. Recent studies show that a wide range of metabolic pathways are controlled by circadian rhythms whose oscillation is affected by nutritional challenges, underscoring the importance of assessing a temporal window for clinical testing and thereby questioning the accuracy of the reading of critical pathological markers in circulation. We have been interested in studying the communication between peripheral tissues under metabolic homeostasis perturbation. Here we present a comparative circadian metabolomic analysis on serum and liver in mice under high fat diet. Our data reveal that the nutritional challenge induces a loss of serum metabolite rhythmicity compared with liver, indicating a circadian misalignment between the tissues analyzed. Importantly, our results show that the levels of serum metabolites do not reflect the circadian liver metabolic signature or the effect of nutritional challenge. This notion reveals the possibility that misleading reads of metabolites in circulation may result in misdiagnosis and improper treatments. Our findings also demonstrate a tissue-specific and time-dependent disruption of metabolic homeostasis in response to altered nutrition.
Keywords: circadian rhythm; liver; metabolism; metabolomics; serum.
© 2016 by The American Society for Biochemistry and Molecular Biology, Inc.
Figures
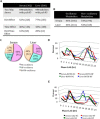
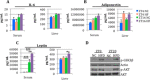
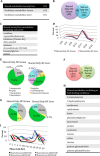
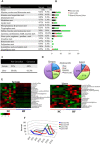
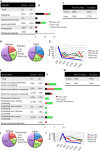
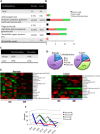
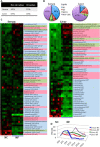
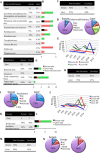
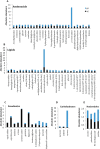
Similar articles
-
Purine Catabolism Shows a Dampened Circadian Rhythmicity in a High-fat Diet-Induced Mouse Model of Obesity.Molecules. 2019 Dec 10;24(24):4524. doi: 10.3390/molecules24244524. Molecules. 2019. PMID: 31835615 Free PMC article.
-
Atlas of Circadian Metabolism Reveals System-wide Coordination and Communication between Clocks.Cell. 2018 Sep 6;174(6):1571-1585.e11. doi: 10.1016/j.cell.2018.08.042. Cell. 2018. PMID: 30193114 Free PMC article.
-
High-fat diet delays and fasting advances the circadian expression of adiponectin signaling components in mouse liver.Endocrinology. 2009 Jan;150(1):161-8. doi: 10.1210/en.2008-0944. Epub 2008 Sep 18. Endocrinology. 2009. PMID: 18801899
-
Circadian Metabolomics in Time and Space.Front Neurosci. 2017 Jul 11;11:369. doi: 10.3389/fnins.2017.00369. eCollection 2017. Front Neurosci. 2017. PMID: 28744188 Free PMC article. Review.
-
Diurnal regulation of lipid metabolism and applications of circadian lipidomics.J Genet Genomics. 2014 May 20;41(5):231-50. doi: 10.1016/j.jgg.2014.04.001. Epub 2014 Apr 21. J Genet Genomics. 2014. PMID: 24894351 Review.
Cited by
-
Quantification of microenvironmental metabolites in murine cancers reveals determinants of tumor nutrient availability.Elife. 2019 Apr 16;8:e44235. doi: 10.7554/eLife.44235. Elife. 2019. PMID: 30990168 Free PMC article.
-
Rosiglitazone reverses high fat diet-induced changes in BMAL1 function in muscle, fat, and liver tissue in mice.Int J Obes (Lond). 2019 Mar;43(3):567-580. doi: 10.1038/s41366-018-0090-5. Epub 2018 May 24. Int J Obes (Lond). 2019. PMID: 29795456 Free PMC article.
-
Untargeted and Targeted Circadian Metabolomics Using Liquid Chromatography-Tandem Mass Spectrometry (LC-MS/MS) and Flow Injection-Electrospray Ionization-Tandem Mass Spectrometry (FIA-ESI-MS/MS).Methods Mol Biol. 2022;2482:311-327. doi: 10.1007/978-1-0716-2249-0_21. Methods Mol Biol. 2022. PMID: 35610436
-
Leptin Signaling in Liver Tissue of a Transgenic Breast Cancer Mouse Model.Cureus. 2020 Jan 22;12(1):e6737. doi: 10.7759/cureus.6737. Cureus. 2020. PMID: 32133259 Free PMC article.
-
Circadian and Sleep Metabolomics Across Species.J Mol Biol. 2020 May 29;432(12):3578-3610. doi: 10.1016/j.jmb.2020.04.027. Epub 2020 May 3. J Mol Biol. 2020. PMID: 32376454 Free PMC article. Review.
References
-
- Dibner C., Schibler U., and Albrecht U. (2010) The mammalian circadian timing system: organization and coordination of central and peripheral clocks. Annu. Rev. Physiol. 72, 517–549 - PubMed
Publication types
MeSH terms
Substances
Grants and funding
LinkOut - more resources
Full Text Sources
Medical