Structure and function of ER membrane contact sites with other organelles
- PMID: 26627931
- PMCID: PMC5117888
- DOI: 10.1038/nrm.2015.8
Structure and function of ER membrane contact sites with other organelles
Abstract
The endoplasmic reticulum (ER) is the largest organelle in the cell, and its functions have been studied for decades. The past several years have provided novel insights into the existence of distinct domains between the ER and other organelles, known as membrane contact sites (MCSs). At these contact sites, organelle membranes are closely apposed and tethered, but do not fuse. Here, various protein complexes can work in concert to perform specialized functions such as binding, sensing and transferring molecules, as well as engaging in organelle biogenesis and dynamics. This Review describes the structure and functions of MCSs, primarily focusing on contacts of the ER with mitochondria and endosomes.
Conflict of interest statement
statement The authors declare no competing interests.
Figures
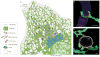
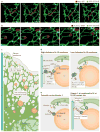
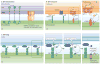
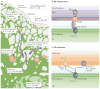
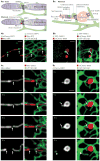
Similar articles
-
Here, there, and everywhere: The importance of ER membrane contact sites.Science. 2018 Aug 3;361(6401):eaan5835. doi: 10.1126/science.aan5835. Science. 2018. PMID: 30072511 Free PMC article. Review.
-
Mitochondrial lipid transport at a glance.J Cell Sci. 2013 Dec 1;126(Pt 23):5317-23. doi: 10.1242/jcs.134130. Epub 2013 Nov 4. J Cell Sci. 2013. PMID: 24190879 Review.
-
ER membranes exhibit phase behavior at sites of organelle contact.Proc Natl Acad Sci U S A. 2020 Mar 31;117(13):7225-7235. doi: 10.1073/pnas.1910854117. Epub 2020 Mar 16. Proc Natl Acad Sci U S A. 2020. PMID: 32179693 Free PMC article.
-
Molecular machineries and physiological relevance of ER-mediated membrane contacts.Theranostics. 2021 Jan 1;11(2):974-995. doi: 10.7150/thno.51871. eCollection 2021. Theranostics. 2021. PMID: 33391516 Free PMC article.
-
Lipids at membrane contact sites: cell signaling and ion transport.EMBO Rep. 2017 Nov;18(11):1893-1904. doi: 10.15252/embr.201744331. Epub 2017 Oct 13. EMBO Rep. 2017. PMID: 29030479 Free PMC article. Review.
Cited by
-
Integrating Transcriptomics and Free Fatty Acid Profiling Analysis Reveal Cu Induces Shortened Lifespan and Increased Fat Accumulation and Oxidative Damage in C. elegans.Oxid Med Cell Longev. 2022 Aug 16;2022:5297342. doi: 10.1155/2022/5297342. eCollection 2022. Oxid Med Cell Longev. 2022. PMID: 36017239 Free PMC article.
-
Molecular Perspectives of Mitochondrial Adaptations and Their Role in Cardiac Proteostasis.Front Physiol. 2020 Aug 27;11:1054. doi: 10.3389/fphys.2020.01054. eCollection 2020. Front Physiol. 2020. PMID: 32982788 Free PMC article. Review.
-
De novo sphingolipid biosynthesis necessitates detoxification in cancer cells.Cell Rep. 2022 Sep 27;40(13):111415. doi: 10.1016/j.celrep.2022.111415. Cell Rep. 2022. PMID: 36170811 Free PMC article.
-
Using Optical Tweezers to Characterize Physical Tethers at Membrane Contact Sites: Grab It, Pull It, Set It Free?Front Cell Dev Biol. 2016 Mar 29;4:22. doi: 10.3389/fcell.2016.00022. eCollection 2016. Front Cell Dev Biol. 2016. PMID: 27066480 Free PMC article. No abstract available.
-
Selenoprotein N is an endoplasmic reticulum calcium sensor that links luminal calcium levels to a redox activity.Proc Natl Acad Sci U S A. 2020 Sep 1;117(35):21288-21298. doi: 10.1073/pnas.2003847117. Epub 2020 Aug 17. Proc Natl Acad Sci U S A. 2020. PMID: 32817544 Free PMC article.
References
-
- Fawcett DW. The Cell. W. B. Saunders; 1981.
-
- Ogata T, Yamasaki Y. Ultra-high-resolution scanning electron microscopy of mitochondria and sarcoplasmic reticulum arrangement in human red, white, and intermediate muscle fibers. Anat Rec. 1997;248:214–223. - PubMed
-
- Shibata Y, Voeltz GK, Rapoport TA. Rough sheets and smooth tubules. Cell. 2006;126:435–439. - PubMed
Publication types
MeSH terms
Substances
Grants and funding
LinkOut - more resources
Full Text Sources
Other Literature Sources
Molecular Biology Databases
Miscellaneous