Detailed mechanistic investigation into the S-nitrosation of cysteamine
- PMID: 26594054
- PMCID: PMC4651662
- DOI: 10.1139/v2012-051
Detailed mechanistic investigation into the S-nitrosation of cysteamine
Abstract
The nitrosation of cysteamine (H2NCH2CH2SH) to produce cysteamine-S-nitrosothiol (CANO) was studied in slightly acidic medium by using nitrous acid prepared in situ. The stoichiometry of the reaction was H2NCH2CH2SH + HNO2 → H2NCH2CH2SNO + H2O. On prolonged standing, the nitrosothiol decomposed quantitatively to yield the disulfide, cystamine: 2H2NCH2CH2SNO → H2NCH2CH2S-SCH2CH2NH2 + 2NO. NO2 and N2O3 are not the primary nitrosating agents, since their precursor (NO) was not detected during the nitrosation process. The reaction is first order in nitrous acid, thus implicating it as the major nitrosating agent in mildly acidic pH conditions. Acid catalyzes nitrosation after nitrous acid has saturated, implicating the protonated nitrous acid species, the nitrosonium cation (NO+) as a contributing nitrosating species in highly acidic environments. The acid catalysis at constant nitrous acid concentrations suggests that the nitrosonium cation nitrosates at a much higher rate than nitrous acid. Bimolecular rate constants for the nitrosation of cysteamine by nitrous acid and by the nitrosonium cation were deduced to be 17.9 ± 1.5 (mol/L)-1 s-1 and 6.7 × 104 (mol/L)-1 s-1, respectively. Both Cu(I) and Cu(II) ions were effective catalysts for the formation and decomposition of the cysteamine nitrosothiol. Cu(II) ions could catalyze the nitrosation of cysteamine in neutral conditions, whereas Cu(I) could only catalyze in acidic conditions. Transnitrosation kinetics of CANO with glutathione showed the formation of cystamine and the mixed disulfide with no formation of oxidized glutathione (GSSG). The nitrosation reaction was satisfactorily simulated by a simple reaction scheme involving eight reactions.
Keywords: cysteamine; kinetics; nitric oxide; nitrosation; thiols.
Figures
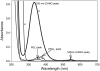
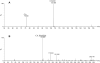
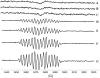
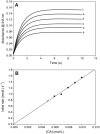
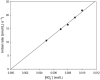
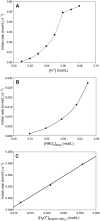
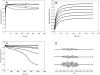
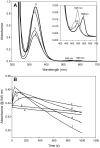
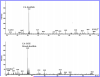
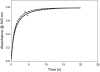
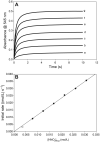
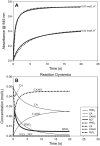
Similar articles
-
Modulation of homocysteine toxicity by S-nitrosothiol formation: a mechanistic approach.J Phys Chem B. 2010 Aug 5;114(30):9894-904. doi: 10.1021/jp103679v. J Phys Chem B. 2010. PMID: 20666529
-
Formation and stability of a nitric oxide donor: S-nitroso-N-acetylpenicillamine.J Phys Chem B. 2006 Mar 16;110(10):5052-61. doi: 10.1021/jp0531107. J Phys Chem B. 2006. PMID: 16526748
-
Kinetics of S-nitrosation of thiols in nitric oxide solutions.Chem Res Toxicol. 1996 Sep;9(6):988-93. doi: 10.1021/tx960036y. Chem Res Toxicol. 1996. PMID: 8870986
-
Catalysis and inhibition of N-nitrosation reactions.IARC Sci Publ. 1984;(57):263-74. IARC Sci Publ. 1984. PMID: 6398294 Review.
-
How are nitrosothiols formed de novo in vivo?Arch Biochem Biophys. 2017 Mar 1;617:137-144. doi: 10.1016/j.abb.2016.10.015. Epub 2016 Oct 26. Arch Biochem Biophys. 2017. PMID: 27794428 Review.
Cited by
-
Cu∥-loaded polydopamine coatings with in situ nitric oxide generation function for improved hemocompatibility.Regen Biomater. 2020 Mar;7(2):153-160. doi: 10.1093/rb/rbz043. Epub 2020 Jan 17. Regen Biomater. 2020. PMID: 32296534 Free PMC article.
-
Cysteamine, an Endogenous Aminothiol, and Cystamine, the Disulfide Product of Oxidation, Increase Pseudomonas aeruginosa Sensitivity to Reactive Oxygen and Nitrogen Species and Potentiate Therapeutic Antibiotics against Bacterial Infection.Infect Immun. 2018 May 22;86(6):e00947-17. doi: 10.1128/IAI.00947-17. Print 2018 Jun. Infect Immun. 2018. PMID: 29581193 Free PMC article.
-
Rapid and selective quantitative colourimetric analysis of nitrite in water using a S-Nitrosothiol based method.Water Res X. 2024 Oct 11;25:100265. doi: 10.1016/j.wroa.2024.100265. eCollection 2024 Dec 1. Water Res X. 2024. PMID: 39497788 Free PMC article.
References
-
- Möller MN, Li Q, Vitturi DA, Robinson JM, Lancaster JR, Jr, Denicola A. Chem. Res. Toxicol. 2007;20(4):709. doi:10.1021/tx700010h. - PubMed
-
- Li H, Samouilov A, Liu X, Zweier JL. J. Biol. Chem. 2001;276(27):24482. doi:10.1074/jbc.M011648200. - PubMed
-
- Godber BL, Doel JJ, Sapkota GP, Blake DR, Stevens CR, Eisenthal R, Harrison R. J. Biol. Chem. 2000;275(11):7757. doi:10.1074/jbc.275.11.7757. - PubMed
Grants and funding
LinkOut - more resources
Full Text Sources
Other Literature Sources
Miscellaneous