Structural basis for suppression of hypernegative DNA supercoiling by E. coli topoisomerase I
- PMID: 26490962
- PMCID: PMC4678816
- DOI: 10.1093/nar/gkv1073
Structural basis for suppression of hypernegative DNA supercoiling by E. coli topoisomerase I
Abstract
Escherichia coli topoisomerase I has an essential function in preventing hypernegative supercoiling of DNA. A full length structure of E. coli topoisomerase I reported here shows how the C-terminal domains bind single-stranded DNA (ssDNA) to recognize the accumulation of negative supercoils in duplex DNA. These C-terminal domains of E. coli topoisomerase I are known to interact with RNA polymerase, and two flexible linkers within the C-terminal domains may assist in the movement of the ssDNA for the rapid removal of transcription driven negative supercoils. The structure has also unveiled for the first time how the 4-Cys zinc ribbon domain and zinc ribbon-like domain bind ssDNA with primarily π-stacking interactions. This novel structure, in combination with new biochemical data, provides important insights into the mechanism of genome regulation by type IA topoisomerases that is essential for life, as well as the structures of homologous type IA TOP3α and TOP3β from higher eukaryotes that also have multiple 4-Cys zinc ribbon domains required for their physiological functions.
© The Author(s) 2015. Published by Oxford University Press on behalf of Nucleic Acids Research.
Figures
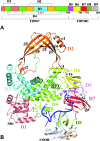
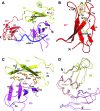
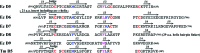
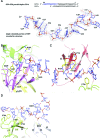
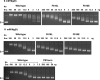
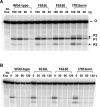
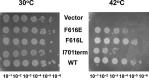
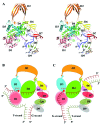
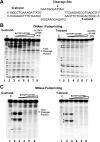
Similar articles
-
Direct interaction between Escherichia coli RNA polymerase and the zinc ribbon domains of DNA topoisomerase I.J Biol Chem. 2003 Aug 15;278(33):30705-10. doi: 10.1074/jbc.M303403200. Epub 2003 Jun 4. J Biol Chem. 2003. PMID: 12788950
-
The role of the Zn(II) binding domain in the mechanism of E. coli DNA topoisomerase I.BMC Biochem. 2002 May 29;3:13. doi: 10.1186/1471-2091-3-13. BMC Biochem. 2002. PMID: 12052259 Free PMC article.
-
Antimicrobial Susceptibility and SOS-Dependent Increase in Mutation Frequency Are Impacted by Escherichia coli Topoisomerase I C-Terminal Point Mutation.Antimicrob Agents Chemother. 2015 Oct;59(10):6195-202. doi: 10.1128/AAC.00855-15. Epub 2015 Jul 27. Antimicrob Agents Chemother. 2015. PMID: 26248366 Free PMC article.
-
[DNA supercoiling and topoisomerases in Escherichia coli].Rev Latinoam Microbiol. 1995 Jul-Sep;37(3):291-304. Rev Latinoam Microbiol. 1995. PMID: 8850348 Review. Spanish.
-
Structure and mechanism of DNA topoisomerases.Annu Rev Biophys Biomol Struct. 1995;24:185-208. doi: 10.1146/annurev.bb.24.060195.001153. Annu Rev Biophys Biomol Struct. 1995. PMID: 7663114 Review.
Cited by
-
Characterization of molecular interactions between Escherichia coli RNA polymerase and topoisomerase I by molecular simulations.FEBS Lett. 2016 Sep;590(17):2844-51. doi: 10.1002/1873-3468.12321. Epub 2016 Aug 4. FEBS Lett. 2016. PMID: 27448274 Free PMC article.
-
Deacetylation of topoisomerase I is an important physiological function of E. coli CobB.Nucleic Acids Res. 2017 May 19;45(9):5349-5358. doi: 10.1093/nar/gkx250. Nucleic Acids Res. 2017. PMID: 28398568 Free PMC article.
-
Crystal structure of the 65-kilodalton amino-terminal fragment of DNA topoisomerase I from the gram-positive model organism Streptococcus mutans.Biochem Biophys Res Commun. 2019 Aug 20;516(2):333-338. doi: 10.1016/j.bbrc.2019.06.034. Epub 2019 Jun 14. Biochem Biophys Res Commun. 2019. PMID: 31204053 Free PMC article.
-
Topoisomerase I Essentiality, DnaA-Independent Chromosomal Replication, and Transcription-Replication Conflict in Escherichia coli.J Bacteriol. 2021 Aug 9;203(17):e0019521. doi: 10.1128/JB.00195-21. Epub 2021 Aug 9. J Bacteriol. 2021. PMID: 34124945 Free PMC article.
-
Restoring Global Gene Regulation through Experimental Evolution Uncovers a NAP (Nucleoid-Associated Protein)-Like Behavior of Crp/Cap.mBio. 2021 Oct 26;12(5):e0202821. doi: 10.1128/mBio.02028-21. Epub 2021 Oct 26. mBio. 2021. PMID: 34700380 Free PMC article.
References
-
- Chen S.H., Chan N.L., Hsieh T.S. New mechanistic and functional insights into DNA topoisomerases. Annu. Rev. Biochem. 2013;82:139–170. - PubMed
-
- Drolet M. Growth inhibition mediated by excess negative supercoiling: The interplay between transcription elongation, R-loop formation and DNA topology. Mol. Microbiol. 2006;59:723–730. - PubMed
-
- Drolet M., Broccoli S., Rallu F., Hraiky C., Fortin C., Masse E., Baaklini I. The problem of hypernegative supercoiling and R-loop formation in transcription. Front. Biosci. 2003;8:d210–d221. - PubMed
Publication types
MeSH terms
Substances
Associated data
- Actions
Grants and funding
LinkOut - more resources
Full Text Sources
Other Literature Sources
Research Materials
Miscellaneous