All-Optical Interrogation of Neural Circuits
- PMID: 26468193
- PMCID: PMC4604230
- DOI: 10.1523/JNEUROSCI.2916-15.2015
All-Optical Interrogation of Neural Circuits
Abstract
There have been two recent revolutionary advances in neuroscience: First, genetically encoded activity sensors have brought the goal of optical detection of single action potentials in vivo within reach. Second, optogenetic actuators now allow the activity of neurons to be controlled with millisecond precision. These revolutions have now been combined, together with advanced microscopies, to allow "all-optical" readout and manipulation of activity in neural circuits with single-spike and single-neuron precision. This is a transformational advance that will open new frontiers in neuroscience research. Harnessing the power of light in the all-optical approach requires coexpression of genetically encoded activity sensors and optogenetic probes in the same neurons, as well as the ability to simultaneously target and record the light from the selected neurons. It has recently become possible to combine sensors and optical strategies that are sufficiently sensitive and cross talk free to enable single-action-potential sensitivity and precision for both readout and manipulation in the intact brain. The combination of simultaneous readout and manipulation from the same genetically defined cells will enable a wide range of new experiments as well as inspire new technologies for interacting with the brain. The advances described in this review herald a future where the traditional tools used for generations by physiologists to study and interact with the brain-stimulation and recording electrodes-can largely be replaced by light. We outline potential future developments in this field and discuss how the all-optical strategy can be applied to solve fundamental problems in neuroscience.
Significance statement: This review describes the nexus of dramatic recent developments in optogenetic probes, genetically encoded activity sensors, and novel microscopies, which together allow the activity of neural circuits to be recorded and manipulated entirely using light. The optical and protein engineering strategies that form the basis of this "all-optical" approach are now sufficiently advanced to enable single-neuron and single-action potential precision for simultaneous readout and manipulation from the same functionally defined neurons in the intact brain. These advances promise to illuminate many fundamental challenges in neuroscience, including transforming our search for the neural code and the links between neural circuit activity and behavior.
Keywords: calcium imaging; genetically encoded calcium sensor; genetically encoded voltage sensor; optogenetics; two-photon microscopy; wavefront shaping.
Copyright © 2015 the authors 0270-6474/15/3513917-10$15.00/0.
Figures
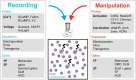
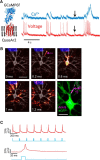
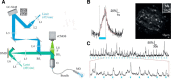
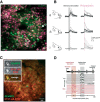
Similar articles
-
Imaging Voltage in Genetically Defined Neuronal Subpopulations with a Cre Recombinase-Targeted Hybrid Voltage Sensor.J Neurosci. 2017 Sep 20;37(38):9305-9319. doi: 10.1523/JNEUROSCI.1363-17.2017. Epub 2017 Aug 23. J Neurosci. 2017. PMID: 28842412 Free PMC article.
-
Simultaneous all-optical manipulation and recording of neural circuit activity with cellular resolution in vivo.Nat Methods. 2015 Feb;12(2):140-6. doi: 10.1038/nmeth.3217. Epub 2014 Dec 22. Nat Methods. 2015. PMID: 25532138 Free PMC article.
-
Let there be light: zebrafish neurobiology and the optogenetic revolution.Rev Neurosci. 2011;22(1):121-30. doi: 10.1515/RNS.2011.013. Rev Neurosci. 2011. PMID: 21615266 Review.
-
Single-trial imaging of spikes and synaptic potentials in single neurons in brain slices with genetically encoded hybrid voltage sensor.J Neurophysiol. 2015 Feb 15;113(4):1249-59. doi: 10.1152/jn.00691.2014. Epub 2014 Nov 19. J Neurophysiol. 2015. PMID: 25411462 Free PMC article.
-
Targeting neurons and photons for optogenetics.Nat Neurosci. 2013 Jul;16(7):805-15. doi: 10.1038/nn.3427. Epub 2013 Jun 25. Nat Neurosci. 2013. PMID: 23799473 Free PMC article. Review.
Cited by
-
Intact skull chronic windows for mesoscopic wide-field imaging in awake mice.J Neurosci Methods. 2016 Jul 15;267:141-9. doi: 10.1016/j.jneumeth.2016.04.012. Epub 2016 Apr 19. J Neurosci Methods. 2016. PMID: 27102043 Free PMC article.
-
Modulating cell signalling in vivo with magnetic nanotransducers.Nat Rev Methods Primers. 2022;2:92. doi: 10.1038/s43586-022-00170-2. Epub 2022 Nov 17. Nat Rev Methods Primers. 2022. PMID: 38111858 Free PMC article.
-
How many neurons are sufficient for perception of cortical activity?Elife. 2020 Oct 26;9:e58889. doi: 10.7554/eLife.58889. Elife. 2020. PMID: 33103656 Free PMC article.
-
Two-photon calcium imaging of neuronal activity.Nat Rev Methods Primers. 2022;2(1):67. doi: 10.1038/s43586-022-00147-1. Epub 2022 Sep 1. Nat Rev Methods Primers. 2022. PMID: 38124998 Free PMC article.
-
Electro-plasmonic nanoantenna: A nonfluorescent optical probe for ultrasensitive label-free detection of electrophysiological signals.Sci Adv. 2019 Oct 18;5(10):eaav9786. doi: 10.1126/sciadv.aav9786. eCollection 2019 Oct. Sci Adv. 2019. PMID: 31667339 Free PMC article.
References
-
- Akerboom J, Carreras Calderón N, Tian L, Wabnig S, Prigge M, Tolö J, Gordus A, Orger MB, Severi KE, Macklin JJ, Patel R, Pulver SR, Wardill TJ, Fischer E, Schüler C, Chen TW, Sarkisyan KS, Marvin JS, Bargmann CI, Kim DS, et al. Genetically encoded calcium indicators for multi-color neural activity imaging and combination with optogenetics. Front Mol Neurosci. 2013;6:2. doi: 10.3389/fnmol.2013.00002. - DOI - PMC - PubMed
Publication types
MeSH terms
Grants and funding
LinkOut - more resources
Full Text Sources
Other Literature Sources