α-Arrestins participate in cargo selection for both clathrin-independent and clathrin-mediated endocytosis
- PMID: 26459639
- PMCID: PMC4712785
- DOI: 10.1242/jcs.175372
α-Arrestins participate in cargo selection for both clathrin-independent and clathrin-mediated endocytosis
Abstract
Clathrin-mediated endocytosis (CME) is a well-studied mechanism to internalize plasma membrane proteins; however, to endocytose such cargo, most eukaryotic cells also use alternative clathrin-independent endocytic (CIE) pathways, which are less well characterized. The budding yeast Saccharomyces cerevisiae, a widely used model for studying CME, was recently shown to have a CIE pathway that requires the GTPase Rho1, the formin Bni1, and their regulators. Nevertheless, in both yeast and mammalian cells, the mechanisms underlying cargo selection in CME and CIE are only beginning to be understood. For CME in yeast, particular α-arrestins contribute to recognition of specific cargos and promote their ubiquitylation by recruiting the E3 ubiquitin protein ligase Rsp5. Here, we show that the same α-arrestin-cargo pairs promote internalization through the CIE pathway by interacting with CIE components. Notably, neither expression of Rsp5 nor its binding to α-arrestins is required for CIE. Thus, α-arrestins are important for cargo selection in both the CME and CIE pathways, but function by distinct mechanisms in each pathway.
Keywords: Internalization; Plasma membrane; Protein trafficking; Ubiquitin ligase; Yeast.
© 2015. Published by The Company of Biologists Ltd.
Conflict of interest statement
The authors declare no competing or financial interests.
Figures
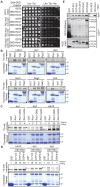
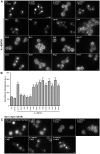
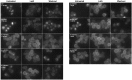
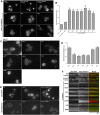
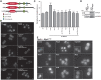
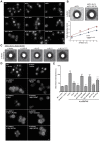
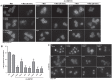
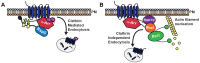
Similar articles
-
Internalization of Heterologous Sugar Transporters by Endogenous α-Arrestins in the Yeast Saccharomyces cerevisiae.Appl Environ Microbiol. 2016 Nov 21;82(24):7074-7085. doi: 10.1128/AEM.02148-16. Print 2016 Dec 15. Appl Environ Microbiol. 2016. PMID: 27694235 Free PMC article.
-
Differential Phosphorylation Provides a Switch to Control How α-Arrestin Rod1 Down-regulates Mating Pheromone Response in Saccharomyces cerevisiae.Genetics. 2016 May;203(1):299-317. doi: 10.1534/genetics.115.186122. Epub 2016 Feb 26. Genetics. 2016. PMID: 26920760 Free PMC article.
-
Syp1 regulates the clathrin-mediated and clathrin-independent endocytosis of multiple cargo proteins through a novel sorting motif.Mol Biol Cell. 2017 Sep 1;28(18):2434-2448. doi: 10.1091/mbc.E15-10-0731. Epub 2017 Jul 12. Mol Biol Cell. 2017. PMID: 28701344 Free PMC article.
-
The α-arrestin family of ubiquitin ligase adaptors links metabolism with selective endocytosis.Biol Cell. 2021 Apr;113(4):183-219. doi: 10.1111/boc.202000137. Epub 2021 Feb 1. Biol Cell. 2021. PMID: 33314196 Review.
-
Mechanisms of Carrier Formation during Clathrin-Independent Endocytosis.Trends Cell Biol. 2018 Mar;28(3):188-200. doi: 10.1016/j.tcb.2017.11.004. Epub 2017 Dec 11. Trends Cell Biol. 2018. PMID: 29241687 Review.
Cited by
-
Toxicity and assimilation of cellulosic copper nanoparticles require α-arrestins in S. cerevisiae.Metallomics. 2023 Mar 6;15(3):mfad011. doi: 10.1093/mtomcs/mfad011. Metallomics. 2023. PMID: 36841230 Free PMC article.
-
Alpha-arrestins Aly1/Art6 and Aly2/Art3 regulate trafficking of the glycerophosphoinositol transporter Git1 and impact phospholipid homeostasis.Biol Cell. 2022 Jan;114(1):3-31. doi: 10.1111/boc.202100007. Epub 2021 Oct 8. Biol Cell. 2022. PMID: 34562280 Free PMC article.
-
A Convergent Functional Genomics Analysis to Identify Biological Regulators Mediating Effects of Creatine Supplementation.Nutrients. 2021 Jul 23;13(8):2521. doi: 10.3390/nu13082521. Nutrients. 2021. PMID: 34444681 Free PMC article.
-
Yeast α-arrestin Art2 is the key regulator of ubiquitylation-dependent endocytosis of plasma membrane vitamin B1 transporters.PLoS Biol. 2019 Oct 28;17(10):e3000512. doi: 10.1371/journal.pbio.3000512. eCollection 2019 Oct. PLoS Biol. 2019. PMID: 31658248 Free PMC article.
-
TORC1 Signaling Controls the Stability and Function of α-Arrestins Aly1 and Aly2.Biomolecules. 2022 Mar 31;12(4):533. doi: 10.3390/biom12040533. Biomolecules. 2022. PMID: 35454122 Free PMC article.
References
-
- Aguilar R. C., Longhi S. A., Shaw J. D., Yeh L.-Y., Kim S., Schön A., Freire E., Hsu A., McCormick W. K., Watson H. A. et al. (2006). Epsin N-terminal homology domains perform an essential function regulating Cdc42 through binding Cdc42 GTPase-activating proteins. Proc. Natl. Acad. Sci. USA 103, 4116-4121. 10.1073/pnas.0510513103 - DOI - PMC - PubMed
-
- Alvaro C. G., O'Donnell A. F., Prosser D. C., Augustine A. A., Goldman A., Brodsky J. L., Cyert M. S., Wendland B. and Thorner J. (2014). Specific alpha-arrestins negatively regulate saccharomyces cerevisiae pheromone response by down-modulating the G-protein-coupled receptor Ste2. Mol. Cell. Biol. 34, 2660-2681. 10.1128/MCB.00230-14 - DOI - PMC - PubMed
Publication types
MeSH terms
Substances
Grants and funding
LinkOut - more resources
Full Text Sources
Other Literature Sources
Molecular Biology Databases