Cryo-electron microscopy structure of the Slo2.2 Na(+)-activated K(+) channel
- PMID: 26436452
- PMCID: PMC4886347
- DOI: 10.1038/nature14958
Cryo-electron microscopy structure of the Slo2.2 Na(+)-activated K(+) channel
Abstract
Na(+)-activated K(+) channels are members of the Slo family of large conductance K(+) channels that are widely expressed in the brain, where their opening regulates neuronal excitability. These channels fulfil a number of biological roles and have intriguing biophysical properties, including conductance levels that are ten times those of most other K(+) channels and gating sensitivity to intracellular Na(+). Here we present the structure of a complete Na(+)-activated K(+) channel, chicken Slo2.2, in the Na(+)-free state, determined by cryo-electron microscopy at a nominal resolution of 4.5 ångströms. The channel is composed of a large cytoplasmic gating ring, in which resides the Na(+)-binding site and a transmembrane domain that closely resembles voltage-gated K(+) channels. In the structure, the cytoplasmic domain adopts a closed conformation and the ion conduction pore is also closed. The structure reveals features that can explain the unusually high conductance of Slo channels and how contraction of the cytoplasmic gating ring closes the pore.
Figures
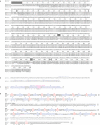
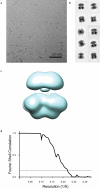

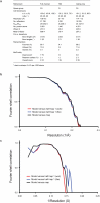
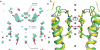
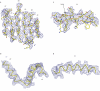
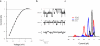
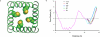
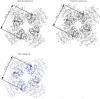
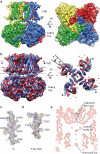
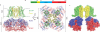
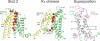
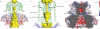


Similar articles
-
Molecular mechanisms of Slo2 K+ channel closure.J Physiol. 2017 Apr 1;595(7):2321-2336. doi: 10.1113/JP273225. Epub 2016 Dec 2. J Physiol. 2017. PMID: 27682982 Free PMC article.
-
Structural basis of human Slo2.2 channel gating and modulation.Cell Rep. 2023 Aug 29;42(8):112858. doi: 10.1016/j.celrep.2023.112858. Epub 2023 Jul 25. Cell Rep. 2023. PMID: 37494189
-
Structural Titration of Slo2.2, a Na+-Dependent K+ Channel.Cell. 2017 Jan 26;168(3):390-399.e11. doi: 10.1016/j.cell.2016.12.030. Epub 2017 Jan 19. Cell. 2017. PMID: 28111072 Free PMC article.
-
Emerging issues of connexin channels: biophysics fills the gap.Q Rev Biophys. 2001 Aug;34(3):325-472. doi: 10.1017/s0033583501003705. Q Rev Biophys. 2001. PMID: 11838236 Review.
-
Structure and function of cardiac potassium channels.Cardiovasc Res. 1999 May;42(2):377-90. doi: 10.1016/s0008-6363(99)00071-1. Cardiovasc Res. 1999. PMID: 10533574 Review.
Cited by
-
Membrane protein isolation and structure determination in cell-derived membrane vesicles.Proc Natl Acad Sci U S A. 2023 May 2;120(18):e2302325120. doi: 10.1073/pnas.2302325120. Epub 2023 Apr 25. Proc Natl Acad Sci U S A. 2023. PMID: 37098056 Free PMC article.
-
Molecular mechanisms of Slo2 K+ channel closure.J Physiol. 2017 Apr 1;595(7):2321-2336. doi: 10.1113/JP273225. Epub 2016 Dec 2. J Physiol. 2017. PMID: 27682982 Free PMC article.
-
Importance of the Choice of a Recombinant System to Produce Large Amounts of Functional Membrane Protein hERG.Int J Mol Sci. 2019 Jun 28;20(13):3181. doi: 10.3390/ijms20133181. Int J Mol Sci. 2019. PMID: 31261773 Free PMC article.
-
Voltage- and calcium-gated ion channels of neurons in the vertebrate retina.Prog Retin Eye Res. 2019 Sep;72:100760. doi: 10.1016/j.preteyeres.2019.05.001. Epub 2019 May 10. Prog Retin Eye Res. 2019. PMID: 31078724 Free PMC article. Review.
-
Stimulation of Slack K(+) Channels Alters Mass at the Plasma Membrane by Triggering Dissociation of a Phosphatase-Regulatory Complex.Cell Rep. 2016 Aug 30;16(9):2281-8. doi: 10.1016/j.celrep.2016.07.024. Epub 2016 Aug 18. Cell Rep. 2016. PMID: 27545877 Free PMC article.
References
-
- Hille B. Ionic channels of excitable membranes. 2nd edn Sinauer Associates; 1992.
-
- Bader CR, Bernheim L, Bertrand D. Sodium-activated potassium current in cultured avian neurones. Nature. 1985;317:540–542. - PubMed
-
- Haimann C, Bader CR. Sodium-activated potassium channel in avian sensory neurons. Cell Biol Int Rep. 1989;13:1133–1139. - PubMed
-
- Kameyama M, et al. Intracellular Na+ activates a K+ channel in mammalian cardiac cells. Nature. 1984;309:354–356. - PubMed
References for Methods section
-
- Webster SM, Del Camino D, Dekker JP, Yellen G. Intracellular gate opening in Shaker K+ channels defined by high-affinity metal bridges. Nature. 2004;428:864–868. doi:10.1038/nature02468. - PubMed
-
- Mastronarde DN. Automated electron microscope tomography using robust prediction of specimen movements. J Struct Biol. 2005;152:36–51. doi:10.1016/j.jsb.2005.07.007. - PubMed
-
- Ludtke SJ, Baldwin PR, Chiu W. EMAN: semiautomated software for high-resolution single-particle reconstructions. J Struct Biol. 1999;128:82–97. doi:10.1006/jsbi.1999.4174. - PubMed
Publication types
MeSH terms
Substances
Associated data
- Actions
- Actions
- Actions
Grants and funding
LinkOut - more resources
Full Text Sources
Other Literature Sources
Molecular Biology Databases