STIM1 dimers undergo unimolecular coupling to activate Orai1 channels
- PMID: 26399906
- PMCID: PMC4598629
- DOI: 10.1038/ncomms9395
STIM1 dimers undergo unimolecular coupling to activate Orai1 channels
Abstract
The endoplasmic reticulum (ER) Ca(2+) sensor, STIM1, becomes activated when ER-stored Ca(2+) is depleted and translocates into ER-plasma membrane junctions where it tethers and activates Orai1 Ca(2+) entry channels. The dimeric STIM1 protein contains a small STIM-Orai-activating region (SOAR)--the minimal sequence sufficient to activate Orai1 channels. Since SOAR itself is a dimer, we constructed SOAR concatemer-dimers and introduced mutations at F394, which is critical for Orai1 coupling and activation. The F394H mutation in both SOAR monomers completely blocks dimer function, but F394H introduced in only one of the dimeric SOAR monomers has no effect on Orai1 binding or activation. This reveals an unexpected unimolecular coupling between STIM1 and Orai1 and argues against recent evidence suggesting dimeric interaction between STIM1 and two adjacent Orai1 channel subunits. The model predicts that STIM1 dimers may be involved in crosslinking between Orai1 channels with implications for the kinetics and localization of Orai1 channel opening.
Figures
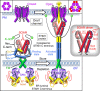
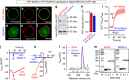
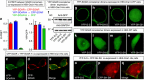
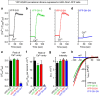
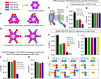
Similar articles
-
Cross-linking of Orai1 channels by STIM proteins.Proc Natl Acad Sci U S A. 2018 Apr 10;115(15):E3398-E3407. doi: 10.1073/pnas.1720810115. Epub 2018 Mar 26. Proc Natl Acad Sci U S A. 2018. PMID: 29581306 Free PMC article.
-
The STIM-Orai Pathway: Conformational Coupling Between STIM and Orai in the Activation of Store-Operated Ca2+ Entry.Adv Exp Med Biol. 2017;993:83-98. doi: 10.1007/978-3-319-57732-6_5. Adv Exp Med Biol. 2017. PMID: 28900910 Free PMC article. Review.
-
Assessing the Molecular Nature of the STIM1/Orai1 Coupling Interface Using FRET Approaches.In: Kozak JA, Putney JW Jr, editors. Calcium Entry Channels in Non-Excitable Cells. Boca Raton (FL): CRC Press/Taylor & Francis; 2018. Chapter 7. In: Kozak JA, Putney JW Jr, editors. Calcium Entry Channels in Non-Excitable Cells. Boca Raton (FL): CRC Press/Taylor & Francis; 2018. Chapter 7. PMID: 30299648 Free Books & Documents. Review.
-
Potent functional uncoupling between STIM1 and Orai1 by dimeric 2-aminodiphenyl borinate analogs.Cell Calcium. 2014 Dec;56(6):482-92. doi: 10.1016/j.ceca.2014.10.005. Epub 2014 Oct 23. Cell Calcium. 2014. PMID: 25459299 Free PMC article.
-
Single-molecule analysis of diffusion and trapping of STIM1 and Orai1 at endoplasmic reticulum-plasma membrane junctions.Mol Biol Cell. 2014 Nov 5;25(22):3672-85. doi: 10.1091/mbc.E14-06-1107. Epub 2014 Jul 23. Mol Biol Cell. 2014. PMID: 25057023 Free PMC article.
Cited by
-
Mapping of the FGF14:Nav1.6 complex interface reveals FLPK as a functionally active peptide modulating excitability.Physiol Rep. 2020 Jul;8(14):e14505. doi: 10.14814/phy2.14505. Physiol Rep. 2020. PMID: 32671946 Free PMC article.
-
Identification of molecular determinants that govern distinct STIM2 activation dynamics.PLoS Biol. 2018 Nov 16;16(11):e2006898. doi: 10.1371/journal.pbio.2006898. eCollection 2018 Nov. PLoS Biol. 2018. PMID: 30444880 Free PMC article.
-
Cross-linking of Orai1 channels by STIM proteins.Proc Natl Acad Sci U S A. 2018 Apr 10;115(15):E3398-E3407. doi: 10.1073/pnas.1720810115. Epub 2018 Mar 26. Proc Natl Acad Sci U S A. 2018. PMID: 29581306 Free PMC article.
-
A focus on extracellular Ca2+ entry into skeletal muscle.Exp Mol Med. 2017 Sep 15;49(9):e378. doi: 10.1038/emm.2017.208. Exp Mol Med. 2017. PMID: 28912570 Free PMC article. Review.
-
Cross-talk between N-terminal and C-terminal domains in stromal interaction molecule 2 (STIM2) determines enhanced STIM2 sensitivity.J Biol Chem. 2019 Apr 19;294(16):6318-6332. doi: 10.1074/jbc.RA118.006801. Epub 2019 Mar 1. J Biol Chem. 2019. PMID: 30824535 Free PMC article.
References
-
- Kar P., Nelson C. & Parekh A. B. CRAC Channels drive digital activation and provide analog control and synergy to Ca2+-dependent gene regulation. Curr. Biol. 22, 242–247 (2012). - PubMed
Publication types
MeSH terms
Substances
Grants and funding
LinkOut - more resources
Full Text Sources
Other Literature Sources
Miscellaneous