Distinct Functions for Anterograde and Retrograde Sorting of SORLA in Amyloidogenic Processes in the Brain
- PMID: 26377460
- PMCID: PMC6795211
- DOI: 10.1523/JNEUROSCI.0427-15.2015
Distinct Functions for Anterograde and Retrograde Sorting of SORLA in Amyloidogenic Processes in the Brain
Abstract
SORLA is a neuronal sorting receptor implicated both in sporadic and familial forms of AD. SORLA reduces the amyloidogenic burden by two mechanisms, either by rerouting internalized APP molecules from endosomes to the trans-Golgi network (TGN) to prevent proteolytic processing or by directing newly produced Aβ to lysosomes for catabolism. Studies in cell lines suggested that the interaction of SORLA with cytosolic adaptors retromer and GGA is required for receptor sorting to and from the TGN. However, the relevance of anterograde or retrograde trafficking for SORLA activity in vivo remained largely unexplored. Here, we generated mouse models expressing SORLA variants lacking binding sites for GGA or retromer to query this concept in the brain. Disruption of retromer binding resulted in a retrograde-sorting defect with accumulation of SORLA in endosomes and depletion from the TGN, and in an overall enhanced APP processing. In contrast, disruption of the GGA interaction did not impact APP processing but caused increased brain Aβ levels, a mechanism attributed to a defect in anterograde lysosomal targeting of Aβ. Our findings substantiated the significance of adaptor-mediated sorting for SORLA activities in vivo, and they uncovered that anterograde and retrograde sorting paths may serve discrete receptor functions in amyloidogenic processes.
Significance statement: SORLA is a sorting receptor that directs target proteins to distinct intracellular compartments in neurons. SORLA has been identified as a genetic risk factor for sporadic, but recently also for familial forms of AD. To confirm the relevance of SORLA sorting for AD processes in the brain, we generated mouse lines, which express trafficking mutants instead of the wild-type form of this receptor. Studying neuronal activities in these mutant mice, we dissected distinct trafficking routes for SORLA guided by two cytosolic adaptors termed GGA and retromer. We show that these sorting pathways serve discrete functions in control of amyloidogenic processes and may represent unique therapeutic targets to interfere with specific aspects of neurodegenerative processes in the diseased brain.
Keywords: APP processing; SORLA; VPS10P domain receptors; adaptors; protein transport; retromer.
Copyright © 2015 the authors 0270-6474/15/3512703-11$15.00/0.
Figures
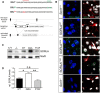
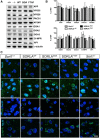
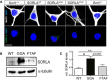
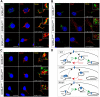
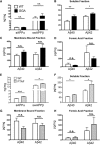
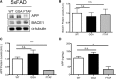
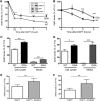
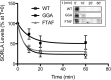
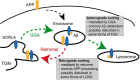
Similar articles
-
SORLA-dependent and -independent functions for PACS1 in control of amyloidogenic processes.Mol Cell Biol. 2013 Nov;33(21):4308-20. doi: 10.1128/MCB.00628-13. Epub 2013 Sep 3. Mol Cell Biol. 2013. PMID: 24001769 Free PMC article.
-
Sorting receptor SORLA--a trafficking path to avoid Alzheimer disease.J Cell Sci. 2013 Jul 1;126(Pt 13):2751-60. doi: 10.1242/jcs.125393. Epub 2013 Jun 26. J Cell Sci. 2013. PMID: 23813966 Review.
-
Retromer binds the FANSHY sorting motif in SorLA to regulate amyloid precursor protein sorting and processing.J Neurosci. 2012 Jan 25;32(4):1467-80. doi: 10.1523/JNEUROSCI.2272-11.2012. J Neurosci. 2012. PMID: 22279231 Free PMC article.
-
SorLA/LR11 regulates processing of amyloid precursor protein via interaction with adaptors GGA and PACS-1.J Biol Chem. 2007 Nov 9;282(45):32956-64. doi: 10.1074/jbc.M705073200. Epub 2007 Sep 12. J Biol Chem. 2007. PMID: 17855360
-
The Role of SORL1 in Alzheimer's Disease.Mol Neurobiol. 2015;51(3):909-18. doi: 10.1007/s12035-014-8742-5. Epub 2014 May 16. Mol Neurobiol. 2015. PMID: 24833601 Review.
Cited by
-
A familial missense variant in the Alzheimer's Disease gene SORL1 impairs its maturation and endosomal sorting.bioRxiv [Preprint]. 2023 Nov 13:2023.07.01.547348. doi: 10.1101/2023.07.01.547348. bioRxiv. 2023. Update in: Acta Neuropathol. 2024 Jan 20;147(1):20. doi: 10.1007/s00401-023-02670-1. PMID: 37461597 Free PMC article. Updated. Preprint.
-
Sorting Out the Role of the Sortilin-Related Receptor 1 in Alzheimer's Disease.J Alzheimers Dis Rep. 2020 May 2;4(1):123-140. doi: 10.3233/ADR-200177. J Alzheimers Dis Rep. 2020. PMID: 32587946 Free PMC article. Review.
-
Pharmacological modulation of autophagy for Alzheimer's disease therapy: Opportunities and obstacles.Acta Pharm Sin B. 2022 Apr;12(4):1688-1706. doi: 10.1016/j.apsb.2021.12.009. Epub 2021 Dec 18. Acta Pharm Sin B. 2022. PMID: 35847516 Free PMC article. Review.
-
Acute GARP depletion disrupts vesicle transport, leading to severe defects in sorting, secretion, and O-glycosylation.bioRxiv [Preprint]. 2024 Oct 14:2024.10.07.617053. doi: 10.1101/2024.10.07.617053. bioRxiv. 2024. PMID: 39416116 Free PMC article. Preprint.
-
The adaptor protein PICK1 targets the sorting receptor SorLA.Mol Brain. 2022 Feb 19;15(1):18. doi: 10.1186/s13041-022-00903-0. Mol Brain. 2022. PMID: 35183222 Free PMC article.
References
-
- Andersen OM, Reiche J, Schmidt V, Gotthardt M, Spoelgen R, Behlke J, von Arnim CA, Breiderhoff T, Jansen P, Wu X, Bales KR, Cappai R, Masters CL, Gliemann J, Mufson EJ, Hyman BT, Paul SM, Nykjaer A, Willnow TE. Neuronal sorting protein-related receptor sorLA/LR11 regulates processing of the amyloid precursor protein. Proc Natl Acad Sci U S A. 2005;28:13461–13466. - PMC - PubMed
-
- Caglayan S, Takagi-Niidome S, Liao F, Carlo AS, Schmidt V, Burgert T, Kitago Y, Füchtbauer EM, Füchtbauer A, Holtzman DM, Takagi J, Willnow TE. Lysosomal sorting of amyloid-beta by the SORLA receptor is impaired by a familial Alzheimer's disease mutation. Sci Transl Med. 2014;6:223ra20. doi: 10.1126/scitranslmed.3007747. - DOI - PubMed
-
- Fjorback AW, Seaman M, Gustafsen C, Mehmedbasic A, Gokool S, Wu C, Militz D, Schmidt V, Madsen P, Nyengaard JR, Willnow TE, Christensen EI, Mobley WB, Nykjaer A, Andersen OM. Retromer binds the FANSHY sorting motif in SorLA to regulate Amyloid Precursor Protein sorting and processing. J Neurosci. 2012;32:1467–1480. doi: 10.1523/JNEUROSCI.2272-11.2012. - DOI - PMC - PubMed
Publication types
MeSH terms
Substances
LinkOut - more resources
Full Text Sources
Other Literature Sources
Molecular Biology Databases
Miscellaneous