Roles of helix H69 of 23S rRNA in translation initiation
- PMID: 26324939
- PMCID: PMC4577190
- DOI: 10.1073/pnas.1507703112
Roles of helix H69 of 23S rRNA in translation initiation
Abstract
Initiation of translation involves the assembly of a ribosome complex with initiator tRNA bound to the peptidyl site and paired to the start codon of the mRNA. In bacteria, this process is kinetically controlled by three initiation factors--IF1, IF2, and IF3. Here, we show that deletion of helix H69 (∆H69) of 23S rRNA allows rapid 50S docking without concomitant IF3 release and virtually eliminates the dependence of subunit joining on start codon identity. Despite this, overall accuracy of start codon selection, based on rates of formation of elongation-competent 70S ribosomes, is largely uncompromised in the absence of H69. Thus, the fidelity function of IF3 stems primarily from its interplay with initiator tRNA rather than its anti-subunit association activity. While retaining fidelity, ∆H69 ribosomes exhibit much slower rates of overall initiation, due to the delay in IF3 release and impedance of an IF3-independent step, presumably initiator tRNA positioning. These findings clarify the roles of H69 and IF3 in the mechanism of translation initiation and explain the dominant lethal phenotype of the ∆H69 mutation.
Keywords: IF2; IF3; fMet-tRNA; ribosome; start codon selection.
Conflict of interest statement
The authors declare no conflict of interest.
Figures
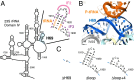
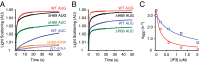
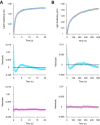
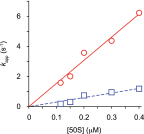
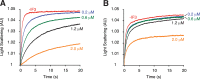
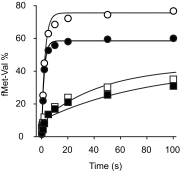
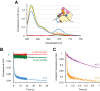
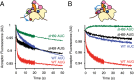
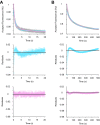
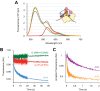
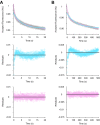
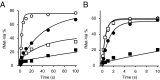
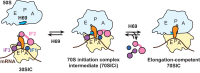
Similar articles
-
IF2 and unique features of initiator tRNAfMet help establish the translational reading frame.RNA Biol. 2018;15(4-5):604-613. doi: 10.1080/15476286.2017.1379636. Epub 2017 Nov 13. RNA Biol. 2018. PMID: 28914580 Free PMC article.
-
Characterization of 16S rRNA mutations that decrease the fidelity of translation initiation.RNA. 2007 Dec;13(12):2348-55. doi: 10.1261/rna.715307. Epub 2007 Oct 17. RNA. 2007. PMID: 17942743 Free PMC article.
-
Direct monitoring of initiation factor dynamics through formation of 30S and 70S translation-initiation complexes on a quartz crystal microbalance.Chemistry. 2013 May 17;19(21):6807-16. doi: 10.1002/chem.201203502. Epub 2013 Mar 27. Chemistry. 2013. PMID: 23536416
-
Initiation of mRNA translation in bacteria: structural and dynamic aspects.Cell Mol Life Sci. 2015 Nov;72(22):4341-67. doi: 10.1007/s00018-015-2010-3. Epub 2015 Aug 11. Cell Mol Life Sci. 2015. PMID: 26259514 Free PMC article. Review.
-
A structural view of translation initiation in bacteria.Cell Mol Life Sci. 2009 Feb;66(3):423-36. doi: 10.1007/s00018-008-8416-4. Cell Mol Life Sci. 2009. PMID: 19011758 Free PMC article. Review.
Cited by
-
IF2 and unique features of initiator tRNAfMet help establish the translational reading frame.RNA Biol. 2018;15(4-5):604-613. doi: 10.1080/15476286.2017.1379636. Epub 2017 Nov 13. RNA Biol. 2018. PMID: 28914580 Free PMC article.
-
The initiation factor 3 (IF3) residues interacting with initiator tRNA elbow modulate the fidelity of translation initiation and growth fitness in Escherichia coli.Nucleic Acids Res. 2022 Nov 11;50(20):11712-11726. doi: 10.1093/nar/gkac1053. Nucleic Acids Res. 2022. PMID: 36399509 Free PMC article.
-
Ribosomes lacking bS21 gain function to regulate protein synthesis in Flavobacterium johnsoniae.Nucleic Acids Res. 2023 Feb 28;51(4):1927-1942. doi: 10.1093/nar/gkad047. Nucleic Acids Res. 2023. PMID: 36727479 Free PMC article.
-
Translation in Prokaryotes.Cold Spring Harb Perspect Biol. 2018 Sep 4;10(9):a032664. doi: 10.1101/cshperspect.a032664. Cold Spring Harb Perspect Biol. 2018. PMID: 29661790 Free PMC article. Review.
-
Intersubunit Bridges of the Bacterial Ribosome.J Mol Biol. 2016 May 22;428(10 Pt B):2146-64. doi: 10.1016/j.jmb.2016.02.009. Epub 2016 Feb 13. J Mol Biol. 2016. PMID: 26880335 Free PMC article. Review.
References
-
- Milón P, Maracci C, Filonava L, Gualerzi CO, Rodnina MV. Real-time assembly landscape of bacterial 30S translation initiation complex. Nat Struct Mol Biol. 2012;19(6):609–615. - PubMed
-
- Carter AP, et al. Crystal structure of an initiation factor bound to the 30S ribosomal subunit. Science. 2001;291(5503):498–501. - PubMed
-
- Simonetti A, et al. Structure of the 30S translation initiation complex. Nature. 2008;455(7211):416–420. - PubMed
-
- Antoun A, Pavlov MY, Lovmar M, Ehrenberg M. How initiation factors maximize the accuracy of tRNA selection in initiation of bacterial protein synthesis. Mol Cell. 2006;23(2):183–193. - PubMed
Publication types
MeSH terms
Substances
LinkOut - more resources
Full Text Sources
Other Literature Sources
Molecular Biology Databases