Resistance to anticancer vaccination effect is controlled by a cancer cell-autonomous phenotype that disrupts immunogenic phagocytic removal
- PMID: 26314964
- PMCID: PMC4694957
- DOI: 10.18632/oncotarget.4754
Resistance to anticancer vaccination effect is controlled by a cancer cell-autonomous phenotype that disrupts immunogenic phagocytic removal
Erratum in
-
Correction: Resistance to anticancer vaccination effect is controlled by a cancer cell-autonomous phenotype that disrupts immunogenic phagocytic removal.Oncotarget. 2018 Jun 26;9(49):29284. doi: 10.18632/oncotarget.25721. eCollection 2018 Jun 26. Oncotarget. 2018. PMID: 30018752 Free PMC article.
Abstract
Immunogenic cell death (ICD) is a well-established instigator of 'anti-cancer vaccination-effect (AVE)'. ICD has shown considerable preclinical promise, yet there remain subset of cancer patients that fail to respond to clinically-applied ICD inducers. Non-responsiveness to ICD inducers could be explained by the existence of cancer cell-autonomous, anti-AVE resistance mechanisms. However such resistance mechanisms remain poorly investigated. In this study, we have characterized for the first time, a naturally-occurring preclinical cancer model (AY27) that exhibits intrinsic anti-AVE resistance despite treatment with ICD inducers like mitoxantrone or hypericin-photodynamic therapy. Further mechanistic analysis revealed that this anti-AVE resistance was associated with a defect in exposing the important 'eat me' danger signal, surface-calreticulin (ecto-CRT/CALR). In an ICD setting, this defective ecto-CRT further correlated with severely reduced phagocytic clearance of AY27 cells as well as the failure of these cells to activate AVE. Defective ecto-CRT in response to ICD induction was a result of low endogenous CRT protein levels (i.e. CRTlow-phenotype) in AY27 cells. Exogenous reconstitution of ecto-rCRT (recombinant-CRT) improved the phagocytic removal of ICD inducer-treated AY27 cells, and importantly, significantly increased their AVE-activating ability. Moreover, we found that a subset of cancer patients of various cancer-types indeed possessed CALRlow or CRTlow-tumours. Remarkably, we found that tumoural CALRhigh-phenotype was predictive of positive clinical responses to therapy with ICD inducers (radiotherapy and paclitaxel) in lung and ovarian cancer patients, respectively. Furthermore, only in the ICD clinical setting, tumoural CALR levels positively correlated with the levels of various phagocytosis-associated genes relevant for phagosome maturation or processing. Thus, we reveal the existence of a cancer cell-autonomous, anti-AVE or anti-ICD resistance mechanism that has profound clinical implications for anticancer immunotherapy and cancer predictive biomarker analysis.
Keywords: calreticulin; immunogenic cell death; patient; predictive biomarker; prognostic biomarker.
Conflict of interest statement
The authors declare that they have no conflict of interest pertaining to this manuscript.
Figures
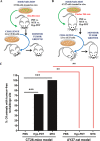
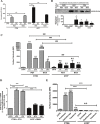
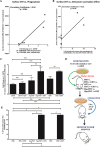
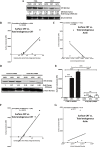
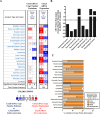
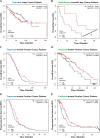
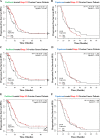
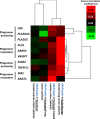
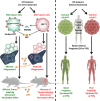
Similar articles
-
ROS-induced autophagy in cancer cells assists in evasion from determinants of immunogenic cell death.Autophagy. 2013 Sep;9(9):1292-307. doi: 10.4161/auto.25399. Epub 2013 Jun 19. Autophagy. 2013. PMID: 23800749
-
Emerging Roles of Calreticulin in Cancer: Implications for Therapy.Curr Protein Pept Sci. 2018 Feb 13;19(4):344-357. doi: 10.2174/1389203718666170111123253. Curr Protein Pept Sci. 2018. PMID: 28079009 Review.
-
Biphasic Increases of Cell Surface Calreticulin Following Treatment with Mitoxantrone.Biol Pharm Bull. 2020 Oct 1;43(10):1595-1599. doi: 10.1248/bpb.b20-00319. Epub 2020 Jul 29. Biol Pharm Bull. 2020. PMID: 32727970
-
Dendritic cell vaccines based on immunogenic cell death elicit danger signals and T cell-driven rejection of high-grade glioma.Sci Transl Med. 2016 Mar 2;8(328):328ra27. doi: 10.1126/scitranslmed.aae0105. Sci Transl Med. 2016. PMID: 26936504
-
Ecto-calreticulin in immunogenic chemotherapy.Immunol Rev. 2007 Dec;220:22-34. doi: 10.1111/j.1600-065X.2007.00567.x. Immunol Rev. 2007. PMID: 17979837 Review.
Cited by
-
EIF2α phosphorylation: a hallmark of both autophagy and immunogenic cell death.Mol Cell Oncol. 2020 Jun 19;7(5):1776570. doi: 10.1080/23723556.2020.1776570. eCollection 2020. Mol Cell Oncol. 2020. PMID: 32944635 Free PMC article.
-
Hypericin in the Light and in the Dark: Two Sides of the Same Coin.Front Plant Sci. 2016 May 6;7:560. doi: 10.3389/fpls.2016.00560. eCollection 2016. Front Plant Sci. 2016. PMID: 27200034 Free PMC article. Review.
-
A Benzenesulfonamide-Based Mitochondrial Uncoupler Induces Endoplasmic Reticulum Stress and Immunogenic Cell Death in Epithelial Ovarian Cancer.Mol Cancer Ther. 2021 Dec;20(12):2398-2409. doi: 10.1158/1535-7163.MCT-21-0396. Epub 2021 Oct 8. Mol Cancer Ther. 2021. PMID: 34625503 Free PMC article.
-
Immunogenic cell death and its therapeutic or prognostic potential in high-grade glioma.Genes Immun. 2022 Feb;23(1):1-11. doi: 10.1038/s41435-021-00161-5. Epub 2022 Jan 19. Genes Immun. 2022. PMID: 35046546 Free PMC article. Review.
-
Preclinical efficacy of immune-checkpoint monotherapy does not recapitulate corresponding biomarkers-based clinical predictions in glioblastoma.Oncoimmunology. 2017 Mar 3;6(4):e1295903. doi: 10.1080/2162402X.2017.1295903. eCollection 2017. Oncoimmunology. 2017. PMID: 28507806 Free PMC article.
References
-
- Krysko DV, Garg AD, Kaczmarek A, Krysko O, Agostinis P, Vandenabeele P. Immunogenic cell death and DAMPs in cancer therapy. Nat Rev Cancer. 2012;12:860–875. - PubMed
Publication types
MeSH terms
Substances
LinkOut - more resources
Full Text Sources
Other Literature Sources
Medical
Research Materials
Miscellaneous