Mammalian target of rapamycin is required for phrenic long-term facilitation following severe but not moderate acute intermittent hypoxia
- PMID: 26224775
- PMCID: PMC4571765
- DOI: 10.1152/jn.00539.2015
Mammalian target of rapamycin is required for phrenic long-term facilitation following severe but not moderate acute intermittent hypoxia
Abstract
Phrenic long-term facilitation (pLTF) is a persistent increase in phrenic nerve activity after acute intermittent hypoxia (AIH). Distinct cell-signaling cascades give rise to pLTF depending on the severity of hypoxemia within hypoxic episodes. Moderate AIH (mAIH; three 5-min episodes, PaO2 ∼35-55 mmHG) elicits pLTF by a serotonin (5-HT)-dependent mechanism that requires new synthesis of brain-derived neurotrophic factor (BDNF), activation of its high-affinity receptor (TrkB), and ERK MAPK signaling. In contrast, severe AIH (sAIH; three 5-min episodes, PaO2 ∼25-30 mmHG) elicits pLTF by an adenosine-dependent mechanism that requires new TrkB synthesis and Akt signaling. Although both mechanisms require spinal protein synthesis, the newly synthesized proteins are distinct, as are the neurochemicals inducing plasticity (serotonin vs. adenosine). In many forms of neuroplasticity, new protein synthesis requires translational regulation via mammalian target of rapamycin (mTOR) signaling. Since Akt regulates mTOR activity, we hypothesized that mTOR activity is necessary for sAIH- but not mAIH-induced pLTF. Phrenic nerve activity in anesthetized, paralyzed, and ventilated rats was recorded before, during, and 60 min after mAIH or sAIH. Rats were pretreated with intrathecal injections of 20% DMSO (vehicle controls) or rapamycin (0.1 mM, 12 μl), a selective mTOR complex 1 inhibitor. Consistent with our hypothesis, rapamycin blocked sAIH- but not mAIH-induced pLTF. Thus spinal mTOR activity is required for adenosine-dependent (sAIH) but not serotonin-dependent (mAIH) pLTF, suggesting that distinct mechanisms regulate new protein synthesis in these forms of spinal neuroplasticity.
Keywords: hypoxia; long-term facilitation; mTOR; motor neuron; phrenic; plasticity; rapamycin; spinal; translational regulation.
Copyright © 2015 the American Physiological Society.
Figures
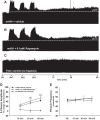
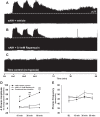
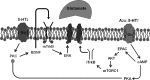
Similar articles
-
Cervical spinal 5-HT2A and 5-HT2B receptors are both necessary for moderate acute intermittent hypoxia-induced phrenic long-term facilitation.J Appl Physiol (1985). 2019 Aug 1;127(2):432-443. doi: 10.1152/japplphysiol.01113.2018. Epub 2019 Jun 20. J Appl Physiol (1985). 2019. PMID: 31219768 Free PMC article.
-
Adenosine-dependent phrenic motor facilitation is inflammation resistant.J Neurophysiol. 2017 Feb 1;117(2):836-845. doi: 10.1152/jn.00619.2016. Epub 2016 Dec 7. J Neurophysiol. 2017. PMID: 27927784 Free PMC article.
-
Mechanisms of severe acute intermittent hypoxia-induced phrenic long-term facilitation.J Neurophysiol. 2021 Apr 1;125(4):1146-1156. doi: 10.1152/jn.00691.2020. Epub 2021 Feb 10. J Neurophysiol. 2021. PMID: 33566744 Free PMC article.
-
Circulatory control of phrenic motor plasticity.Respir Physiol Neurobiol. 2019 Jul;265:19-23. doi: 10.1016/j.resp.2019.01.004. Epub 2019 Jan 11. Respir Physiol Neurobiol. 2019. PMID: 30639504 Free PMC article. Review.
-
NADPH oxidase activity is necessary for acute intermittent hypoxia-induced phrenic long-term facilitation.J Physiol. 2009 May 1;587(Pt 9):1931-42. doi: 10.1113/jphysiol.2008.165597. Epub 2009 Feb 23. J Physiol. 2009. PMID: 19237427 Free PMC article. Review.
Cited by
-
Sustained Hypoxia Elicits Competing Spinal Mechanisms of Phrenic Motor Facilitation.J Neurosci. 2016 Jul 27;36(30):7877-85. doi: 10.1523/JNEUROSCI.4122-15.2016. J Neurosci. 2016. PMID: 27466333 Free PMC article.
-
Cervical spinal 5-HT2A and 5-HT2B receptors are both necessary for moderate acute intermittent hypoxia-induced phrenic long-term facilitation.J Appl Physiol (1985). 2019 Aug 1;127(2):432-443. doi: 10.1152/japplphysiol.01113.2018. Epub 2019 Jun 20. J Appl Physiol (1985). 2019. PMID: 31219768 Free PMC article.
-
Spinal AMP kinase activity differentially regulates phrenic motor plasticity.J Appl Physiol (1985). 2020 Mar 1;128(3):523-533. doi: 10.1152/japplphysiol.00546.2019. Epub 2020 Jan 23. J Appl Physiol (1985). 2020. PMID: 31971473 Free PMC article.
-
Adenosine-dependent phrenic motor facilitation is inflammation resistant.J Neurophysiol. 2017 Feb 1;117(2):836-845. doi: 10.1152/jn.00619.2016. Epub 2016 Dec 7. J Neurophysiol. 2017. PMID: 27927784 Free PMC article.
-
Impact of inflammation on developing respiratory control networks: rhythm generation, chemoreception and plasticity.Respir Physiol Neurobiol. 2020 Mar;274:103357. doi: 10.1016/j.resp.2019.103357. Epub 2019 Dec 30. Respir Physiol Neurobiol. 2020. PMID: 31899353 Free PMC article. Review.
References
-
- Bach KB, Mitchell GS. Hypoxia-induced long-term facilitation of respiratory activity is serotonin dependent. Respir Physiol 104: 251–260, 1996. - PubMed
-
- Baker-Herman TL, Fuller DD, Bavis RW, Zabka AG, Golder FJ, Doperalski NJ, Johnson RA, Watters JJ, Mitchell GS. BDNF is necessary and sufficient for spinal respiratory plasticity following intermittent hypoxia. Nat Neurosci 7: 48–55, 2004. - PubMed
Publication types
MeSH terms
Substances
Grants and funding
LinkOut - more resources
Full Text Sources
Other Literature Sources
Miscellaneous