Interleukin-1 Receptor Type 2 Acts with c-Fos to Enhance the Expression of Interleukin-6 and Vascular Endothelial Growth Factor A in Colon Cancer Cells and Induce Angiogenesis
- PMID: 26209639
- PMCID: PMC4571972
- DOI: 10.1074/jbc.M115.644823
Interleukin-1 Receptor Type 2 Acts with c-Fos to Enhance the Expression of Interleukin-6 and Vascular Endothelial Growth Factor A in Colon Cancer Cells and Induce Angiogenesis
Abstract
Interleukin-1 receptor type 2 (IL1R2) acts as a decoy receptor of exogenous IL-1; however, its intracellular activity is poorly understood. We previously demonstrated that IL1R2 intracellularly activates the expression of several proinflammatory cytokines and affects cell migration. In this study, we found that intracellular IL1R2 expression was increased in human colorectal cancer cells (CRCs) compared with normal colon cells. We also observed that the mRNA levels of IL1R2 were highly correlated with IL-6 in tumor tissues of CRC patients. By modulating its expression in CRC cells, we verified that enhanced IL1R2 expression transcriptionally activated the expression of IL-6 and VEGF-A. Conditioned medium harvested from IL1R2-overexpressing CRC cells contained higher levels of IL-6 and VEGF-A than that from vector control cells and significantly enhanced the proliferation, migration, and tube formation of cultured endothelial cells. We further demonstrated a positive association of intracellular IL1R2 levels with tumor growth and microvessel density in xenograft mouse models. These results revealed that IL1R2 activates the expression of angiogenic factors. Mechanistically, we revealed that IL1R2 complexes with c-Fos and binds to the AP-1 site at the IL-6 and VEGF-A promoters. Together, these results reveal a novel function of intracellular IL1R2 that acts with c-Fos to enhance the transcription of IL-6 and VEGF-A, which promotes angiogenesis in CRC.
Keywords: angiogenesis; c-Fos; colon cancer; interleukin 6 (IL-6); interleukin-1 receptor type 2; vascular endothelial growth factor (VEGF).
© 2015 by The American Society for Biochemistry and Molecular Biology, Inc.
Figures
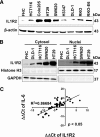
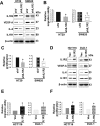
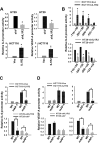
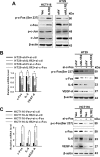
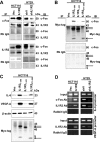
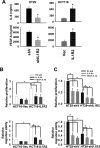
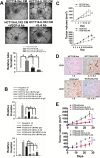
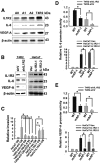
Similar articles
-
Transcription Factor FOSL1 Promotes Angiogenesis of Colon Carcinoma by Regulating the VEGF Pathway Through Activating TIMP1.Biochem Genet. 2024 Oct;62(5):3389-3402. doi: 10.1007/s10528-023-10547-x. Epub 2023 Dec 16. Biochem Genet. 2024. PMID: 38103125
-
Ectopic expression of interleukin-1 receptor type II enhances cell migration through activation of the pre-interleukin 1alpha pathway.Cytokine. 2009 Jan;45(1):32-8. doi: 10.1016/j.cyto.2008.10.013. Epub 2008 Nov 20. Cytokine. 2009. PMID: 19026558
-
miR-23a-3p is a Key Regulator of IL-17C-Induced Tumor Angiogenesis in Colorectal Cancer.Cells. 2020 Jun 1;9(6):1363. doi: 10.3390/cells9061363. Cells. 2020. PMID: 32492770 Free PMC article.
-
NRF2 blockade suppresses colon tumor angiogenesis by inhibiting hypoxia-induced activation of HIF-1α.Cancer Res. 2011 Mar 15;71(6):2260-75. doi: 10.1158/0008-5472.CAN-10-3007. Epub 2011 Jan 28. Cancer Res. 2011. PMID: 21278237
-
Negative regulator IL-1 receptor 2 (IL-1R2) and its roles in immune regulation of autoimmune diseases.Int Immunopharmacol. 2024 Jul 30;136:112400. doi: 10.1016/j.intimp.2024.112400. Epub 2024 Jun 7. Int Immunopharmacol. 2024. PMID: 38850793 Review.
Cited by
-
IL1R2 Blockade Suppresses Breast Tumorigenesis and Progression by Impairing USP15-Dependent BMI1 Stability.Adv Sci (Weinh). 2019 Nov 13;7(1):1901728. doi: 10.1002/advs.201901728. eCollection 2020 Jan. Adv Sci (Weinh). 2019. PMID: 31921558 Free PMC article.
-
C-fos upregulates P-glycoprotein, contributing to the development of multidrug resistance in HEp-2 laryngeal cancer cells with VCR-induced resistance.Cell Mol Biol Lett. 2018 Feb 20;23:6. doi: 10.1186/s11658-017-0067-8. eCollection 2018. Cell Mol Biol Lett. 2018. PMID: 29483928 Free PMC article.
-
Phenotypic and transcriptomic characterization of canine myeloid-derived suppressor cells.Sci Rep. 2019 Mar 5;9(1):3574. doi: 10.1038/s41598-019-40285-3. Sci Rep. 2019. PMID: 30837603 Free PMC article.
-
PKCθ-JunB axis via upregulation of VEGFR3 expression mediates hypoxia-induced pathological retinal neovascularization.Cell Death Dis. 2020 May 7;11(5):325. doi: 10.1038/s41419-020-2522-0. Cell Death Dis. 2020. PMID: 32382040 Free PMC article.
-
Heterorhabditis and Photorhabdus Symbiosis: A Natural Mine of Bioactive Compounds.Front Microbiol. 2022 Mar 29;13:790339. doi: 10.3389/fmicb.2022.790339. eCollection 2022. Front Microbiol. 2022. PMID: 35422783 Free PMC article. Review.
References
Publication types
MeSH terms
Substances
LinkOut - more resources
Full Text Sources
Other Literature Sources
Research Materials