Mapping the SUMOylated landscape
- PMID: 26185901
- PMCID: PMC4869838
- DOI: 10.1111/febs.13378
Mapping the SUMOylated landscape
Abstract
SUMOylation is a post-translational modification that regulates a multitude of cellular processes, including replication, cell-cycle progression, protein transport and the DNA damage response. Similar to ubiquitin, SUMO (small ubiquitin-like modifier) is covalently attached to target proteins in a reversible process via an enzymatic cascade. SUMOylation is essential for nearly all eukaryotic organisms, and deregulation of the SUMO system is associated with human diseases such as cancer and neurodegenerative diseases. Therefore, it is of great interest to understand the regulation and dynamics of this post-translational modification. Within the last decade, mass spectrometry analyses of SUMO proteomes have overcome several obstacles, greatly expanding the number of known SUMO target proteins. In this review, we briefly outline the basic concepts of the SUMO system, and discuss the potential of proteomic approaches to decipher SUMOylation patterns in order to understand the role of SUMO in health and disease.
Keywords: SUMO; cross-talk; group modification; mass spectrometry; post-translational modification; proteomics; site-specific; small ubiquitin-like modifier; ubiquitin.
© The Authors. FEBS Journal published by John Wiley & Sons Ltd on behalf of Federation of European Biochemical Societies.
Figures
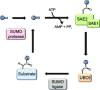
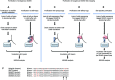
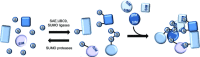
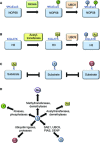
Similar articles
-
The post-translational modification, SUMOylation, and cancer (Review).Int J Oncol. 2018 Apr;52(4):1081-1094. doi: 10.3892/ijo.2018.4280. Epub 2018 Feb 22. Int J Oncol. 2018. PMID: 29484374 Free PMC article. Review.
-
Different proteomic strategies to identify genuine Small Ubiquitin-like MOdifier targets and their modification sites in Trypanosoma brucei procyclic forms.Cell Microbiol. 2015 Oct;17(10):1413-22. doi: 10.1111/cmi.12467. Epub 2015 Jul 24. Cell Microbiol. 2015. PMID: 26096196
-
A comprehensive compilation of SUMO proteomics.Nat Rev Mol Cell Biol. 2016 Sep;17(9):581-95. doi: 10.1038/nrm.2016.81. Epub 2016 Jul 20. Nat Rev Mol Cell Biol. 2016. PMID: 27435506
-
Molecular mechanisms in SUMO conjugation.Biochem Soc Trans. 2020 Feb 28;48(1):123-135. doi: 10.1042/BST20190357. Biochem Soc Trans. 2020. PMID: 31872228 Review.
-
Analysis of changes in SUMO-2/3 modification during breast cancer progression and metastasis.J Proteome Res. 2014 Sep 5;13(9):3905-18. doi: 10.1021/pr500119a. Epub 2014 Aug 7. J Proteome Res. 2014. PMID: 25072996
Cited by
-
Chromatin Targeting of HIPK2 Leads to Acetylation-Dependent Chromatin Decondensation.Front Cell Dev Biol. 2020 Sep 1;8:852. doi: 10.3389/fcell.2020.00852. eCollection 2020. Front Cell Dev Biol. 2020. PMID: 32984337 Free PMC article.
-
SUMOylation inhibition overcomes proteasome inhibitor resistance in multiple myeloma.Blood Adv. 2023 Feb 28;7(4):469-481. doi: 10.1182/bloodadvances.2022007875. Blood Adv. 2023. PMID: 35917568 Free PMC article.
-
Exploring potential targets for natural product therapy of DN: the role of SUMOylation.Front Pharmacol. 2024 Oct 4;15:1432724. doi: 10.3389/fphar.2024.1432724. eCollection 2024. Front Pharmacol. 2024. PMID: 39431155 Free PMC article. Review.
-
Posttranslational modifications in proteins: resources, tools and prediction methods.Database (Oxford). 2021 Apr 7;2021:baab012. doi: 10.1093/database/baab012. Database (Oxford). 2021. PMID: 33826699 Free PMC article.
-
Systems approaches to understand oxygen sensing: how multi-omics has driven advances in understanding oxygen-based signalling.Biochem J. 2022 Feb 11;479(3):245-257. doi: 10.1042/BCJ20210554. Biochem J. 2022. PMID: 35119457 Free PMC article. Review.
References
-
- Jackson SP & Durocher D (2013) Regulation of DNA damage responses by ubiquitin and SUMO. Mol Cell 49, 795–807. - PubMed
-
- Melchior F, Schergaut M & Pichler A (2003) SUMO: ligases, isopeptidases and nuclear pores. Trends Biochem Sci 28, 612–618. - PubMed
-
- Evdokimov E, Sharma P, Lockett SJ, Lualdi M & Kuehn MR (2008) Loss of SUMO1 in mice affects RanGAP1 localization and formation of PML nuclear bodies, but is not lethal as it can be compensated by SUMO2 or SUMO3. J Cell Sci 121, 4106–4113. - PubMed
Publication types
MeSH terms
Substances
Grants and funding
LinkOut - more resources
Full Text Sources
Other Literature Sources